Corals are marine magicians. As colonies of the tiny ocean organisms grow, they transform the calcium that circulates in seawater into enormous limestone reefs. These reefs—which can extend for more than 1,000 miles and provide homes for crabs, eels, sea horses, and other aquatic creatures—are counted among the world’s great natural wonders.
In the late 1960s, a pair of scientists at Pennsylvania State University decided to use a new, high-powered microscope to take a closer look at these remarkable coralline structures. What the two saw would launch an interdisciplinary team on a research odyssey from the shallow, tropical waters of the South Pacific to the modern hospital operating room, where their discovery is now helping surgeons repair patients’ damaged bones.
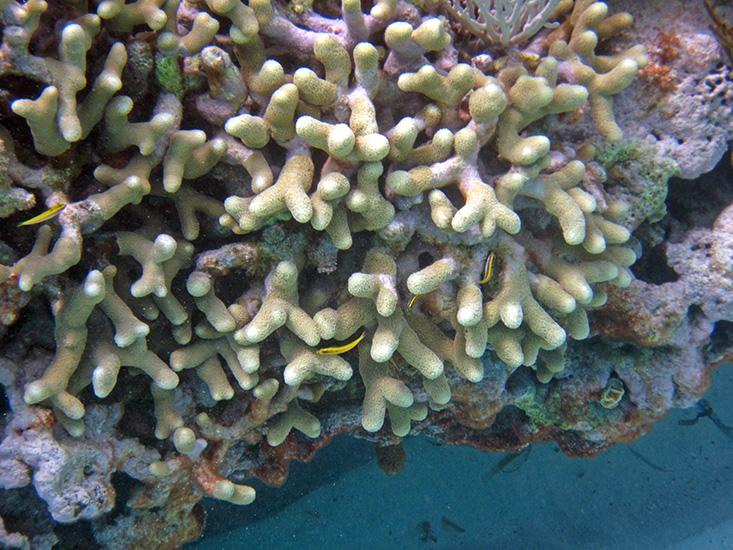
In 2012, the members of that team were given Golden Goose awards, an annual prize initiated by a United States Congressman to honor seemingly offbeat research that has paid unexpected dividends. “It turns out that most of science is still happenstance, and many good discoveries are not planned,” said honoree Rodney White of the serendipity that aided the team’s efforts.
The journey began with Jon Weber, a marine geochemist who specialized in studying the carbonate spines, shells, and skeletons of marine invertebrates, such as starfish, sea urchins, and coral. He collected many of his samples himself, scuba-diving throughout the South Pacific and hauling his specimens back to his lab in Pennsylvania. There, Weber would crush his samples to a fine powder and analyze their chemical makeup. He was curious how the composition of marine skeletons varied across species and whether it was shaped by environmental factors, such as water temperature, salinity, and depth. Weber published a number of papers on these questions throughout the 1960s and early 1970s, but the arrival of a new piece of laboratory equipment sent his research off in an unexpected direction.
In the mid 1960s, Penn State became one of the first institutions in the U.S. to acquire a scanning electron microscope. Traditional microscopes tend to produce flattened, two-dimensional images, but scanning electron microscopes allow scientists to produce micrographs with a large depth of field—and to get a better view of their samples’ three-dimensional structures.
The coral skeletons looked like blocks of three-dimensional mesh, or extremely well aerated Swiss cheese.
“There were a lot of questions that just couldn’t be answered without that kind of equipment,” said Eugene White, a materials scientist who helped set up and run Penn State’s microscope, in an interview before his death last year. “It was the first commercially built electron microscope in this part of the country. It was very popular. People from all around the East Coast would come to see what it could do.”
White worked with these visiting researchers, some of whom were from major companies, getting a close-up view of many manmade materials. A few years after the microscope arrived on campus, White’s friend Jon Weber started showing up at the Material Research Lab with his collection of undersea skeletons. Weber and White put the specimens under the microscope, beginning with sea urchin spines and moving on to corals. They were amazed by what they saw. The coral skeletons were composed of a dense network of pores, entirely shot through with small, interconnected holes. They looked like blocks of three-dimensional mesh, or extremely well aerated Swiss cheese. “We were really fascinated by the physical structure of these corals,” White said. “The structures were so uniquely different from anything we had ever worked with.” The corals didn’t resemble any of the synthetic materials that White had previous examined, but they did look, the researchers eventually realized, an awful lot like human bone.
Though it may seem hard and brittle, bone is living tissue, constantly rebuilding itself. That’s good news, because it means that when bone is damaged, the tissue is capable of regenerating. Although small fractures often heal on their own, bone can’t grow in a void; if a large chunk of bone is missing, surgeons need to implant some sort of scaffold or matrix to which new bone cells can cling.
Bone is naturally porous, and doctors have learned that they can repair bone damage by transplanting small pieces of real human bone into the site of a patient’s defect or injury. New bone tissue then grows into the microscopic channels that crisscross the transplanted bone. These bone grafts have become a mainstay of modern medicine and can be used to mend battlefield injuries, remedy birth defects, heal complex fractures, reduce arthritis pain, and stabilize knee and hip replacements.
But the procedure has drawbacks. Typically, doctors have relied upon two primary sources for transplanted bone; they can harvest a small bit of a patient’s own bone—typically from the hip—or use bone taken from a cadaver. Neither is ideal. Tissue from cadavers can transmit disease, and removing a patient’s own bone is painful and can cause serious complications.
As a result, scientists have long sought alternative materials that might be suitable for bone grafting. Engineers have created some promising candidates, such as porous ceramics, in the lab, but recreating the uniform, completely interconnected pores that characterize human bone has proven daunting. “It’s just very difficult to duplicate what is happening in nature,” said Sridhar Komarneni, a materials scientist at Penn State, and the editor in chief of the Journal of Porous Materials.
Now it seemed that nature itself might provide a solution.
In 1971, White invited his nephew Rodney to spend the summer working in his laboratory. Rodney White, who was then a student at State University of New York Upstate Medical University, collaborated with his uncle and Weber, taking photographs and making molds of coral skeletons. The more that the trio examined the structures, the more they suspected that a mass of tiny, marine invertebrates had managed to do what engineers could not: create an ideal substitute for human bone.
Using a glorified pressure cooker, Della Roy had turned tropical coral into artificial bone.
The pores of the coral skeletons were uniformly sized, evenly distributed, and completely interconnected, which would allow bone and blood cells to flow through the implant and new blood vessels and bone tissue to grow into the graft. Weber, White, and White outlined some of these advantages in a 1972 paper in Science and suggested that Porites coral, often called finger coral, might make a particularly good source of implant material.
“The ability to have a bone substitute in a bottle and take it out and implant it was of great interest at that time,” said Rodney White, now medical director of vascular surgery at the Long Beach Memorial Heart and Vascular Institute.
There was, however, a major problem: Coral skeletons are made of calcium carbonate, which would be absorbed by the human body. “Calcium carbonate was worthless as a material,” Eugene White said. The researchers needed to figure out how to transform the skeleton into a more stable material, without altering its complex structure.
The researchers enlisted the help of Della Roy, a chemist who also worked in the Materials Research Laboratory. She put slices of Porites coral into a tube with a phosphate solution and then heated the tube under high pressure. The phosphate in the solution displaced the carbonate; the sample Roy pulled from the tube hours later was composed of a calcium phosphate compound known as hydroxyapatite, the primary mineral in human bone. The process had not changed the coral’s architecture. Using a glorified pressure cooker, Roy had turned tropical coral into artificial bone.
“That is the key—transforming that carbonate into hydroxyapatite, which is compatible with the body, and preserving the porous structure,” Komarneni said. “It’s a neat idea to transform this.”
Rodney White recruited some surgeons at SUNY to perform animal trials of the new material, which was named coralline hydroxyapatite. The doctors implanted bits of the material into the legs of adult dogs. The coralline implants performed well; new bone slowly formed on the graft and there was no sign of rejection or infection.
A handful of research teams at a variety of different institutions subsequently performed their own studies—in dogs, rabbits, monkeys, and, eventually, humans—with similarly encouraging results. Today, coralline hydroxyapatite has earned a legitimate place in the surgeon’s reconstructive toolbox. It’s used in dental implants, facial reconstruction, spinal fusion, and fracture repair. Tens of thousands of patients have benefitted from it.
“It’s a fascinating material,” said Robert Bucholz, who, before he passed away in 2016, was an orthopedic surgeon at the University of Texas Southwestern Medical Center, and had conducted some of the first human studies. “It was one of the first of the synthetic bone graft substitute materials, and it’s withstood the test of time.”
Emily Anthes is a Brooklyn-based science journalist and author of the book Frankenstein’s Cat: Cuddling Up to Biotech’s Brave New Beasts.
This story was commissioned by the Science Philanthropy Alliance as part of its Science to Society series, illustrating the importance of basic scientific research.