On the morning of Dec. 7, 1979, a 32-year-old Alan Guth woke up with an idea. It had come into his head the previous night, but now, in the light of a California day, he could see the shape of the thing, and was itching to work through the math. He hopped on his bike and rode to his office at the Stanford Linear Accelerator Center. His excitement got him there in record time: 9 minutes, 32 seconds. At his desk, Guth neatly carried out the calculations in his notebook, forming the numbers and symbols in tight, careful lines. Then, at the top of a fresh page, he wrote in all caps: SPECTACULAR REALIZATION.
A year later and some 6,000 miles away, in Moscow, in the middle of the night, Andrei Linde, having read Guth’s paper, had his own spectacular realization. He had been working on his own idea and now he saw how to bring it to life by fixing the difficulties that plagued Guth’s theory. He woke his sleeping wife. “I think I know how the universe was created.”
Guth and Linde had worked out the beginnings of the theory of cosmic inflation. The theory would go through several incarnations over the next few decades, as kinks were worked out and details honed. But the core idea was spectacularly simple: In the earliest fraction of a second of time, a small patch of universe expanded faster than the speed of light, doubling its size again and again, growing a million trillion trillion times bigger in the blink of an eye. A little patch of world, about the size of a dime, grew into our entire observable universe.
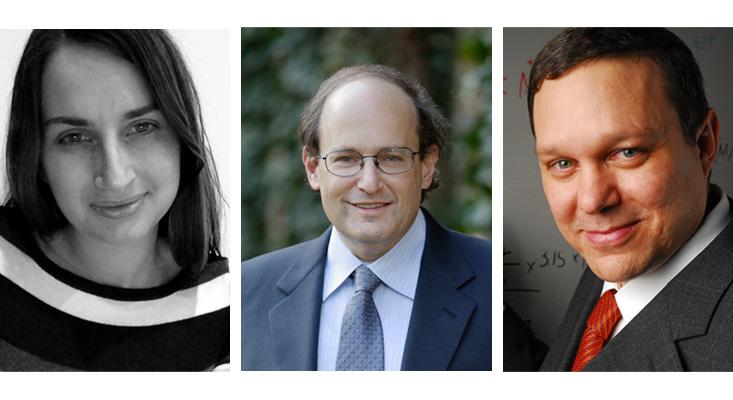
What began as a radical notion has now become standard wisdom among physicists—except, notably, Paul Steinhardt, Anna Ijjas, and Avi Loeb. The three physicists recently wrote a scathing article in Scientific American arguing that it’s time to abandon inflation and look for a competing idea. (What idea, you ask? Steinhardt, conveniently, has one that he’s been pushing for decades.) Inflation is too unlikely to occur, too flexible to be confirmed or rejected experimentally, and too messy in its implications, the threesome argued. It “cannot be evaluated using the scientific method.”
It’s not surprising, then, that Guth and Linde—along with physicists David Kaiser and Yasunori Nomura—published a terse response in Scientific American earlier this month defending their theory. What is more surprising, perhaps, is that 29 more of the world’s leading physicists signed it—including four Nobel laureates and a Field’s medalist.
What is it about this theory that has the greatest minds in the known universe leaping to its defense?
In the media flurry that followed, the disagreement between these groups of physicists was presented as a straight debate, of the kind that often occurs in science when there are multiple interpretations of data. But describing an equivalence between the opinions of Steinhardt, Ijjas, and Loeb on the one hand, and nearly the entire cosmology community on the other, is a mistake.
The long list of signatories to the recent rebuttal letter in Scientific American puts the lie to the claim that the community is divided. When Ed Witten, Steven Weinberg, Leonard Susskind, Frank Wilczek, Juan Maldacena, Eva Silverstein, Sir Martin Rees, and Stephen Hawking (to name a few) write a letter saying you’ve gotten something wrong … well it’s probably worth considering.
The rebuttal letter also challenges us to understand more clearly why so many scientists are passionate about inflation. What is it about this theory that has the greatest minds in the known universe leaping to its defense?
From its inception, inflation has offered a remarkable synthesis of seemingly unrelated aspects of physics. It draws on a concept from particle physics called scalar fields—fields that look the same no matter how you view them, but can contain energy or pressure. Their high level of symmetry suggests that one would be most likely to find them in the earliest moments of the universe’s history, which makes them relevant to cosmology. And scalar fields have the special property that they can have negative pressure—a curiosity that takes on deeper meaning in the context of general relativity, where pressure contributes to the curvature of spacetime. Normal pressure produces gravity. Negative pressure produces inflation.
Inflation shows us that we can take a scalar field from particle physics, apply Einstein’s theory of general relativity, sprinkle in some quantum fluctuations, and get the entire universe replete with all the features we observe when we look around us—features that otherwise would remain inexplicable—from the homogenous distribution of stars and galaxies in all directions and the large-scale geometry of spacetime to the precise bumps and wiggles in the remnant heat from the Big Bang to the very existence of matter itself. When the incredible burst of inflation begins, the uncertainty that rules nature’s tiniest scales is stretched to astronomical proportions, linking the quantum world with the cosmic. When it ends, the expansion energy is transformed into matter and radiation, which sinks into the ripples and divots of spacetime forged by those quantum fluctuations, laying a blueprint for the formation of stars and galaxies.
As Kaiser, the letter’s co-author and a physicist at the Massachusetts Institute of Technology, explains, “Inflation is a conservative, minimalist outcome of two of the most important conceptual principles in physics: the equivalence principle of general relativity and the uncertainty principle of quantum mechanics.” If a theory’s explanatory power, as co-author Nomura of the University of California, Berkeley says, lies in the ratio of the number of things it can explain to the number of assumptions it takes to explain them, inflation packs a serious punch.
You don’t have to show that the universe began easily or quickly. You just have to show that it could begin at all.
To be fair, when Guth and Linde first put forth the idea, there were aspects that seemed a little, well, odd—as if inflation arose by exploiting loopholes in physics. Yes, it’s true nothing can travel through space faster than light, but space itself can expand faster than light; no one had ever seen a scalar field, but physics didn’t expressly forbid it; Einstein himself had conceived of an antigravitational force that would stretch space, but no one had ever observed the stuff. But in the decades since, all of those weird ingredients have shown up. In 1998, astrophysicists discovered an antigravitational force—“dark energy”—that’s driving the accelerated expansion of space right now, with the galaxies farthest from us crossing out of our cosmic horizon faster than the speed of light. In 2012, physicists at the Large Hadron Collider in CERN found clear evidence of a scalar field—the Higgs field. As Murray Gell-Mann once quipped, in physics, “Everything not forbidden is compulsory.” Including, it seems, the universe itself.
In its early days, too, testing inflation seemed nearly impossible. It predicted that the background heat from the early universe that pervades the sky today—stretched into microwave wavelengths now by 13 billion years of cosmic expansion—would be uniform in temperature to 1 part in 100,000, but beyond that, tiny temperature fluctuations would take on a specific statistical distribution across the sky. No one in 1980 could have imagined the minute level of detail with which spacecraft like NASA’s WMAP, launched in 2001, and the ESA’s Planck Satellite, launched in 2009, would map those tiny differences, bearing out the expectations of inflationary models.
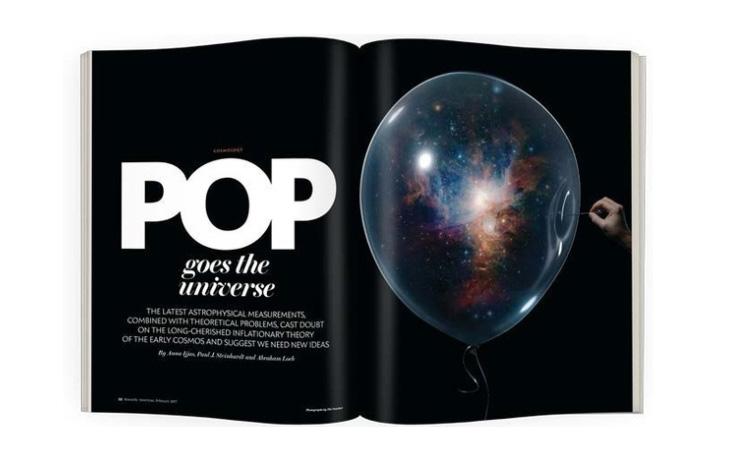
“The most astonishing thing for me is that it has proved so susceptible to experimental-observational confirmation,” says Susskind, the Stanford physicist and founder of string theory who co-signed the letter. “The outlook for further confirmation is excellent and to say otherwise indicates a lack of appreciation for the accomplishments to date.” Ongoing experiments—including telescopes that are now searching the skies for primordial gravitational waves—could further confirm the predictions of inflation.
(Susskind, incidentally, was one of the first people with whom Guth shared his spectacular realization on that fateful December day back in 1979. Susskind listened to his colleague’s jubilant news, then said, “You know, the most amazing thing is that they pay us for this.”)
One of inflation’s early predictions seemed, at the time, flat out wrong. A universe that underwent inflation ought to appear extremely flat (its spacetime metric Euclidean to any measurable degree), because any curvature it had will have been stretched to such huge proportions that you’d never notice it—just like you don’t notice the Earth’s curvature when you’re standing on a flat sidewalk. But the metric of spacetime, according to general relativity, is determined by how much stuff is in it, and a flat metric requires a critical amount of mass and energy. Adding up the weight of all the galaxies in the observable universe, physicists came up about 96 percent short.
That’s not a little wrong—that’s absurdly wrong. And yet, in the days since, astrophysicists have discovered that the universe is full of invisible dark matter, detectable by its gravitational effects, which brought the total up to 27 percent of the critical mass. Then came the discovery of dark energy, which could be weighed by its effect on the expansion of space, bringing the total to a perfect 100 percent—the critical number for a flat universe, far too conspiratorial to be a fluke.
Given all of inflation’s successes, why are Steinhardt, Ijjas and Loeb claiming that it isn’t science?
First, they argue that inflation is too unlikely to occur. The initial conditions required for that scalar field to start inflating, they say, requires an improbable amount of fine-tuning. Within the chaotic conditions at the beginning of time, they argue, you’d need a large, smooth, flat patch of spacetime within which inflation could occur. But large, smooth, flat patches of spacetime are hard to come by—in fact, they are the very features of our universe that inflation was invented to explain. Getting the right initial conditions to trigger inflation, then, would render inflation totally unnecessary.
That might have been true in the past, but it was never clear that it really mattered. If you start with chaos, there’s bound to be a smooth patch in it somewhere, just randomly, and it doesn’t matter how rare it is because once it inflates it grows so big it completely dominates the universe. That’s the thing about cosmology—you don’t have to show that the universe began easily or quickly. You just have to show that it could begin at all.
Theories that can predict anything predict nothing.
In any case, the story has since changed dramatically in the last few years. Major developments1 in what’s known as “full numerical general relativity”—powerful computer simulations of the actual dynamics of spacetime—have shown, surprisingly, that inflation can begin much more easily than anyone ever thought. In fact, you don’t need a smooth, flat patch—even lumpy, messy regions will start inflating. “This has been one of the biggest leaps forward,” Kaiser says.
“The problem of the initial smooth patch has disappeared, in our opinion,” says Leonardo Senatore, a Stanford physicist who worked on the simulations and signed the letter to Scientific American. According to the work, only very few barriers to inflation remain. This “reversed the problem of the probability to start inflation,” says Senatore. “Right now, it seems unlikely for it not to start.” “The problem of initial conditions was solved in 2015,” Linde agrees. “This criticism shows a total ignorance of what is going on.”
So what about the second criticism, that inflation is too flexible to be tested? It’s true that while the idea behind inflation is simple, its parameters can be tweaked in seemingly endless ways: You can change the energy scale at which inflation begins, the features of the scalar field that drives its expansion, or the number of times spacetime doubles in size before inflation rolls to an end. Turning the dial on any of those parameters leads to different patterns in the microwave background radiation, different arrangements of stars and galaxies and different amplitudes of gravitational waves. In other words, the critics say, go out and measure almost anything and someone will say, “hey, that’s evidence for inflation.” Theories that can predict anything predict nothing. Inflation, they say, isn’t science.
But supporters argue that this shows a fundamental misunderstanding of what inflation is. It’s not a single model, they insist—it’s a class of models, a sweeping principle, a paradigm from which individual models can be derived and then tested. The key is to figure out which model of inflation is right—if any—and not to prove or falsify all of them all in one fell swoop. “Each model makes specific predictions, and can be tested with precision by the traditional methods of empirical science,” says Guth, now at MIT. Darwin’s concept of evolution by natural selection does not predict exactly which species of animal you’ll see outside your window; Einstein’s concept that gravity is the curvature of spacetime does not predict the actual spacetime geometry of our universe; and inflation doesn’t predict every last bump and wiggle in the microwave background radiation.
It’s fashionable to refer to the philosopher Karl Popper’s falsification criterion as a kind of litmus test to determine what is and is not science—Susskind has called those who are quick to cry Popper “the Popperazzi.” But in the case of inflation, invoking Popper doesn’t quite add up. “I don’t think that Popper, or any other responsible thinker, has ever advocated that to decide if a theory is scientific, one should ask what class of theories does that theory belong to, and then ask if there is an experimental finding that would rule out that entire class at once,” Guth says. “That would make no sense.”
Philosophers of science have long known that falsification is not as black and white as it sounds. For every theoretical prediction we put to the test, there are scores of auxiliary assumptions implicit in the entire experimental set-up—observations are “theory-laden,” as the philosophers say. If a prediction doesn’t pan out, is it the theory we’re testing that’s wrong, or one of the auxiliary assumptions? They’re impossible to disentangle. Historians of science, meanwhile, know that science has never proceeded by falsification anyway. If a theory has real explanatory power, scientists don’t abandon it just because it seems too flexible or even if it’s disproven by an anomalous observation. They abandon it if a better theory comes along.
You can create a universe from nothing—you can create infinite universes from nothing—as long as they all add up to nothing.
Take Newtonian gravity. It was a remarkably elegant idea—connecting the motions of falling apples with the motions of orbiting planets—but it had its problems. One of them was that Mercury’s orbit did not conform to its equations. Mercury, by Popperazzi standards, had rendered Newtonian gravity falsified. Wash your hands of it, take it out with the trash falsified. But no one tossed the theory aside, nor should they have. It was only when general relativity came along—which could accurately predict Mercury’s orbit, yes, but also had more elegance, more explanatory power—that Newtonian gravity was declared wrong. “Wrong” is too strong a term, really—when velocities are small and gravity is weak, Einstein’s equations reduce to Newton’s. Newtonian gravity was right—it just wasn’t the whole picture.
Inflation produces a remarkably accurate account of the geometry of the observable universe and explains why distant patches of sky that would never otherwise have come into contact are in near perfect thermal equilibrium. Like Newtonian gravity, inflation isn’t the whole picture. It can’t be—because it isn’t formulated using quantum gravity, the complete “theory of everything” that will unify quantum mechanics with general relativity. “In my opinion, the problems with inflation are probably going to be solved by a deeper theory of quantum gravity, or by some other process that occurred before slow roll (non-eternal) inflation started in our patch of the universe,” says co-signer Juan Maldacena of the Institute for Advanced Study. “The problems with the hot Big Bang theory were not removed by discarding the Big Bang theory. They were solved by another type of evolution prior to the big bang phase (namely, inflation). Similarly, I think that the problems with inflation will not be solved by ditching inflation but by some other theory of what happened before.”
And here’s the kicker: Inflationary cosmology itself provides the best hope we have for getting an empirical look at quantum gravity in action. After all, the whole point of inflation is that it takes the quantum-scale dynamics of spacetime itself and magnifies them to cosmic proportions. In doing so, it allows us to study physics at energies 10 billion times greater than the energy scales reached by the Large Hadron Collider at CERN. We will never find a better magnifying glass to peer at the most intricate details of reality. The horizon of a ginormous black hole would make a decent one, but it would still be nothing compared to inflation. “This may be the closest we will ever get to seeing the combined effects of quantum mechanics and general relativity,” Susskind says. One can begin to understand, then, why physicists from fields ranging from the most concrete experimental astrophysics to the most abstract M-theory signed the letter supporting inflation.
Now for the third and final criticism leveraged by Steinhardt, Ijjas, and Loeb. Back in the early days of inflation, Linde realized that thanks to quantum uncertainty, inflation won’t stop at precisely the same time everywhere. So as our universe exits the inflationary phase and begins its ordinary subluminal expansion, a little patch of scalar field remains. That little patch inflates into a whole new universe, which in turn leaves behind yet another patch, creating yet another universe, ad infinitum. Each universe can contain different fields with different particles with different masses with different behavior. Linde called it the eternal self-reproducing universe. Today, physicists call it the multiverse.
The multiverse was yet another aspect of inflation that seemed kind of crazy at first, but then the rest of physics caught up. String theory, for instance, turned out to describe a near-infinite number of universes, all of which cried out for a physical mechanism that might produce them. Meanwhile, observations like the value of dark energy were turning up that seemed improbably fine-tuned for the existence of biological life, crying out for an explanation. In each case, eternal inflation came to the rescue.
Still, not everyone likes the idea of a multiverse. Steinhardt, Ijjas, and Loeb refer to it as “the multimess.” They dislike the fact that various features of physics can differ from one universe to the next, because that reduces the features of our little universe to mere happenstance. “I believe it is a great advantage of the theory,” Linde says, “but they believe it’s a crime.” But not liking the implications of a theory doesn’t make it wrong. “It does seem to make some people uncomfortable—but the comfort zone is not necessarily where we want to be,” Susskind says.
It does seem like universes forever beyond our reach might also lie beyond the reach of experiment. But there’s an interesting way that eternal inflation can be put to the test. Energy is always conserved in physics—you can’t create more stuff than what you started with. And eternal inflation seems, on glance, to violate this with abandon. There’s another loophole, though: Gravity is a form of negative energy. As inflation creates more universes, it simultaneously creates more gravity, and the two work to perfectly cancel out. Energy—which adds up to zero—is conserved. What about other conservation laws? Angular momentum is conserved—but if you tally up the angular momentum of all the galaxies in the universe, it sums to zero. Electric charge is conserved, but the universe is electrically neutral; it adds up to zero, too. Eternal inflation can make universes like ours precisely because it doesn’t have to violate any conservation laws to do it. It’s making more and more of nothing.
Again, inflation has taken what seems like a loophole in physics and exposes it to be a profound glimpse into the nature of reality: You can create a universe from nothing—you can create infinite universes from nothing—as long as they all add up to nothing. Not only is that a deep insight, it also creates a testable prediction. “Eternal inflation certainly predicts that the average density of all conserved quantities should be zero,” Guth says. “So if we ever became convinced that the universe has a nonzero density of electric charge or angular momentum, eternal inflation would no longer be an option.”
Still, infinity makes Steinhardt, Ijjas, and Loeb uneasy because it makes it extremely difficult to define probabilities for the outcomes of measurements. In an infinite multiverse, anything that can happen will happen—an infinite number of times. Such a situation requires what’s called a probability measure to assign different likelihoods to different events, even when there an infinite number of them. But different measures yield different probabilities, and it hasn’t been clear which measure is the right one. That leaves us in a situation where we can’t predict anything, the critics say.
That might be true—but all it means is that physicists have to figure out which measure to use, not that the multiverse is a free-for-all. “Even the rarest of events will occur an infinite number of times,” Guth explains. “But such events will remain extremely rare. The claim that no outcome will be preferred over any other has absolutely no basis in logic. Mathematicians have known for nearly a century that probabilities can be rigorously defined on infinite spaces, and it is certainly not the case that all events must be equally likely. So, unfortunately, even if there is a multiverse, the chances of my winning the Massachusetts lottery tomorrow will remain incredibly small.”
“The problem of the measure is very complicated,” Linde says. “It’s very similar to what happened with the interpretation of quantum mechanics. Everyone knows that quantum mechanics works, but people have been debating its interpretation for 100 years. The multiverse is somewhat similar. It is a deep problem, putting a quantum mechanical description of gravity and cosmology all together.”
A deep problem, indeed—and far subtler than critics of the multiverse imply. (Steinhardt, Ijjas, and Loeb, it should be noted, are far from the only multiverse critics.)The infinite universes that comprise the multiverse are separated from one another by event horizons which, because they are described by both general relativity and quantum mechanics, are strange objects, and not always what they seem. In the last few decades, physicists have discovered that fundamental assumptions about the nature of reality come into question whenever an event horizon lurks. Thought experiments and paradoxes concerning the fate of information that falls behind the horizon of a black hole led Susskind to introduce the notion of “horizon complementarity”: The two sides of a horizon should not be thought of as distinct regions of spacetime, but more like two different descriptions of the very same reality. If horizon complementarity holds in the multiverse, it’s possible that the multiverse is a kind of vast redundancy, and the fundamental theory of the universe may well be written in terms of a single observable universe, rendering the measure problem moot. All the metaphysical baggage of the multiverse, and the distaste some people have for it, might be nothing more than an artifact of all-too-classical thinking. “You have to interpret the multiverse carefully,” says Nomura. “Our world is quantum mechanical.”
Hawking—who signed the letter—has been working on a model he calls top-down cosmology. In his view, in light of quantum mechanics, it doesn’t make sense to talk about the origin and evolution of the universe as if it followed a single unique trajectory. Instead, he argues, we ought to use the universe as we observe it right now—coupled with the assumption that it arose from nothing—and take a quantum superposition of every possible history that could have led from nothing to now. It’s not that we don’t know which history really occurred—it’s that they all occurred. Rather than a multiverse with a single history, you have a single universe with multiple histories. When Hawking takes the sum of these histories to determine the most probable path, it is—voila!—a history in which the early universe went through inflation. Inflation pops out on its own, from a theory that doesn’t involve a multiverse.
Many roads, it seems, lead back to inflation and inflation in turn leads to unexpected places. Steinhardt, Ijjas and Loeb are standing by their criticisms of the theory, and have made a website to reiterate them. But the 33 leading physicists who signed the letter—and countless others—are more confident in inflation than ever, exploring its strange territory, optimistic that it will eventually lead them to its own replacement: a more complete theory of the universe’s origin. That, certainly, is science. And it is pretty spectacular.
Amanda Gefter is a physics writer and author of Trespassing on Einstein’s Lawn: A father, a daughter, the meaning of nothing and the beginning of everything. She lives in Cambridge, Massachusetts.
References
East, W.E., Kleban, W., Linde, A., & Senatore, L. Beginning inflation in an inhomogenous universe. arXiv (2016). Retrieved from DOI: 10.1088/1475-7516/2016/09/010