You might say the brain is our most photogenic organ. We are, thanks to modern neuroimaging, living amid an explosion of brain data. Just consider: We can zoom into the brain’s connectivity to the most minute, molecular level. We can trace individual cells as well as entire cell populations. We can turn neurons on and off just like a light switch. We can even genetically engineer our way through an animal’s memory traces, to play with its behavior. And yet, even with these powers, the way the brain ultimately works eludes us. Some neuroscientists are tempted to see this as an existential crisis for the field.
Still, I wouldn’t say neuroscience is in a crisis. It only seems that way because too much of neuroscience has focused on favorite theories derived from a few pet systems. And this has limited the field’s outlook, quite literally. Take the paradigmatic case of vision. Most of 20th-century brain research has been built on our understanding of how we see. This wasn’t an accident.
What if we broadened our theories to include the workings of less popular parts of the brain?
The captivating beauty of the visual system is its apparent display of logic. The visual system gives us specialized areas in the brain that create and process our perception of particular sensory features. These include orientation, movement, shape, and color. It’s almost as if you can map the physical world onto discrete and orderly neuronal structures.1 This brain-map principle was found in other senses, too, including audition. Tonal frequencies line up in the auditory cortex, similar to the keys of a piano.
Perhaps this feature now proves to be a bug for general theories of brain function. The difficulty in mapping other physiological and mental functions—such as interoception, reward and motivation, or conscious awareness—onto discrete neural structures is that, unlike vision, not all of these activities represent spatial structures by default. They might instead represent a relational measure that connects an organism’s constitution with fluctuating information from the world.
Mapping is, without a doubt, a notable characteristic of many sensory and cognitive systems. However, it might just be one of many material expressions, not the ultimate principle by which the brain organizes its neural activity. What if we broadened our theories to include the workings of less popular parts of the brain?
As I argue in my new book, Smellosophy: What the Nose Tells the Mind, an obvious choice is olfaction. Our sense of smell offers an intriguing challenge to the mapping paradigm in vision. The nose is tailored to measure an unpredictable chemical array in our environment, allowing the brain to evaluate when minute molecular traces shift behavioral meanings to signal pleasure, danger, or novelty.
Ramón y Cajal, a founding father of neuroscience, had already recognized this first when, at the turn of the 20th century, he drew attention to the sense of smell as an exemplary model to learn how the brain makes sense of the world. Though olfaction, to most scientists during Cajal’s time, looked to be a capricious and quirky sense without much sophistication—and so of little interest to studies of cognition and its biological basis—Cajal believed understanding smell would grant us better insight into other sensory systems.
The reason why has to do with an intriguing feature specific to the olfactory system. That is, it takes only two synapses for information to travel from the air, through your nose, and to the core cortex, deep in the brain. To put this into perspective: two synapses won’t get you out of the retina in the visual system. You cannot find a more direct route bringing the brain in touch with the world!
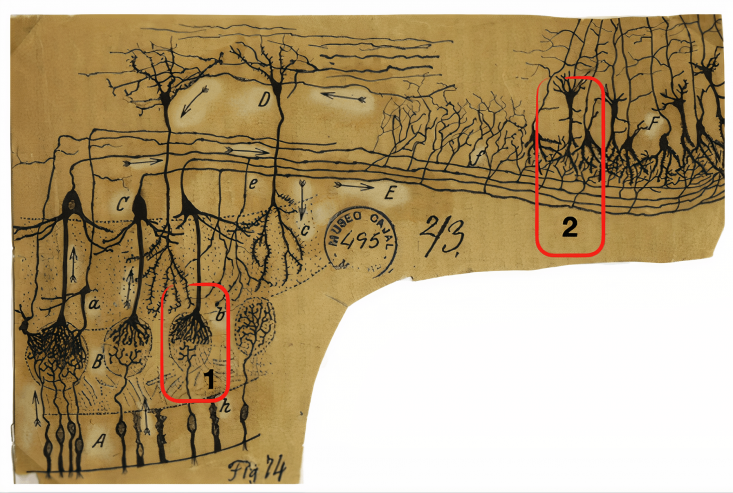
This closeness to the world does not imply that our sense of smell is simple. The complexity of smell is mind-boggling. The chemical composition of your surroundings—and we’re talking hundreds and hundreds of molecules—is changing constantly and rapidly. Your nose picks up volatile airborne compounds that interact with your olfactory receptors (situated on the cilia of the sensory neurons in the nasal epithelium) in a combinatorial fashion. This means that different receptors detect different parts of different molecules, and that one molecule interacts with various receptors via different features. The function of the olfactory system is not to map chemicals discretely in space. Instead, it tracks and computes the statistics of a changing chemical environment: How many, in what concentration, and how often do chemicals co-occur as a molecular cloud? (Our nose is tuned to detect blends of different chemicals—coffee aroma, for example, consists of 800 different compounds.) Simply consider the system’s mind-boggling capacity: If you calculate all possible combinations of structure-receptor interactions in smell (with one molecule hypothetically activating 100 receptors), you’d end up with a number higher than atoms in the galaxy.
Neuroscientists did not pick up Cajal’s advice until the late 20th century.3 There was a certain pragmatism for this. Smell has always been notoriously hard to investigate. As many people can personally attest, the experience of scents is inherently variable. Things can smell different not just between different people but also for the same person.
I wouldn’t say neuroscience is in a crisis. It only seems that way because too much of neuroscience has focused on favorite theories derived from a few pet systems.
When you experience a glass of red wine, for example, you may enjoy its dominant cherry aroma—until someone comments on a strong vanilla note in the same glass of wine. Suddenly the cherry moves to the backseat of your conscious awareness, while the vanilla aroma pops into focus—even though you had not noticed it before. How do you turn such ephemeral and transient nature of odors into a measurable, stable, and comparable object of scientific study?
Linda Buck and Richard Axel set scientists on the path to answering that question with the discovery of the olfactory receptors. The olfactory receptors happened to be the most structurally diverse and sizable member of the largest multigene family of protein receptors in the mammalian genome (so-called G-protein coupled receptors—or GPCRs in short). Their striking diversity and sheer size—olfactory receptor genes have more “genetic storage” than the immune system, occupying about 4 percent of the mammalian genome—caught the attention of scientists interested in mechanisms of gene recombination and the evolutionary diversification of biological processes. How did some biological entities, like proteins, evolve to facilitate a variety of functions? The superfamily of GPCRs orchestrate numerous fundamental biological processes, including vision, the detection of neurotransmitters in the brain, and the regulation of immune responses.
These receptors turned out to be a genetic goldmine for which, along with other work on the organization of the olfactory system, Buck and Axel received the 2004 Nobel Prize in Physiology or Medicine. Olfaction, long the Cinderella of the senses, was thrown into the limelight of the scientific mainstream. As a result, smell emerged as a promising molecular model to think about GPCRs—the target of about half of all drug studies—and the causal principles governing their functional interactions.
For example, how broad is the tuning of these proteins? Do some receptors respond to a wider array of molecular features than others, and might this suggest a genetic difference? How do genetic differences between receptors link to the wiring of the brain (receptor genetics is a fundamental driver in the neural development of the olfactory system)? What’s more, what combinations of molecular features may block or even enhance the response of these proteins? How these proteins execute a vast variety of functions remains a site of ongoing discovery.
With only two synapses straight into the cortex, a full understanding of the olfactory brain looked imminent. Yet the apparent simplicity of the olfactory system soon proved deceptive. Today, three decades after the receptor discovery, the mechanisms of odor perception continue to flummox researchers. Rather than close in on a full understanding, we merely started to appreciate the hidden complexity with which the brain makes sense of scents.
Neuroscientists were wrong to rely on vision as a guide to how the brain works. Arranging smells in a map-like way may sound like a nonstarter. Jasmine scent over here and coriander aroma over there? But this approach soon reveals its limits. By what features would you map potentially more than a trillion odor chemicals in neural space? Are ketones, for instance, placed next to aldehydes, or closer to esters? And where do you put indole, an unpleasant smelling crystalline organic compound found in coal tar and feces?
Recent research on the olfactory system reveals such a visual system-inspired design is mistaken. The olfactory cortex is not organized in a logical spatial manner, like other sensory cortices are. Instead of a map, you face a seemingly random and complicated mosaic of signals. In contrast with color vision and sound, the stimulus in olfaction is multidimensional, not low-dimensional. Color perception is based on the visible spectrum of electromagnetic waves of light and auditory perception is based on the frequency of pressure waves of air, which can be mapped linearly to neural correlates. But odor quality is linked to structurally highly diverse chemicals. These chemicals have about 5,000 molecular features, not correlated in any straightforward manner to odor quality, that olfactory receptors (of which humans have about 400 types) pick up on to identify them.
The brain is not simply a projection screen. It is fundamentally a pattern-recognition device.
How the human brain ought to use neural space to organize this plethora of non-spatial chemical information is, as yet, unresolved. In a way, how your brain processes the complex chemistry of smells is closer to doing mathematics than mapping.
Recall the capacity of combinatorial coding by the receptors. Odors are computed from a widely distributed mosaic of signals: All the brain “sees” (for a lack of a better term) is what receptors light up, how many of them, for how long, and in what combination and ratio. With about 400 types of receptors participating in a combinatorial coding of 5,000 molecular parameters, this turns out to be a rather sophisticated task.
The real question we must ask is: What kind of sensory information is picked up and interpreted via the olfactory system that can be linked to neural correlates? What characterizes olfactory encounters “in the wild” (outside the discrete administration of simple stimuli in the laboratory) is the inherent unpredictability of the chemical stimulus in its environment and its interaction with the sensory interface. This point has implications for our general understanding of how the brain works.
The olfactory system does not require a map mirroring some fixed physical features in the world because its chemical stimulus is constantly in flux. The brain, drawing on memory, recognizes patterns in the chemical composition of the olfactory stimulus. This is one way for the olfactory system to foreground novelty, meaning the introduction of unknown (and potentially behaviorally relevant) compounds into an otherwise constant environment. A similar enough principle of novelty detection in pattern recognition applies to other sensory systems that aren’t strictly mappable, either. Consider interoceptive systems, which regulate processes within an organism like heart rate to hormonal changes.
Paying attention to the brain’s peculiarities can, in other words, be insightful, not counter-productive. Such idiosyncrasies, like the weird complexity and variability of smell, now turn out vital to understanding the brain—how it maneuvers an organism through a landscape of fast-changing molecular combinations. The processing behind this is highly dynamic and radically prompt in answering how, what, and when to choose. The brain is not simply a projection screen. It is fundamentally a pattern-recognition device. Tracking the chemical statistics of an ever-changing environment needs a simple solution to a complex problem—an overly specialized map may even be disadvantageous here. Our brains evolved from our bodies, after all, not the other way around.
Ann-Sophie Barwich is a cognitive scientist and empirical philosopher. She is the author of Smellosophy: What the Nose Tells the Mind. Follow her on Twitter @smellosopher.
Footnotes
1. A series of breakthrough experiments on the visual cortex of the cat, by Torsten Wiesel and David Hubel, cemented the explanatory primacy of a mapping approach to brain function in sensory systems. Hubel and Wiesel’s ideas were informed by prior research on the motor system—Wilder Penfield’s “homunculus” from 1937 (with specific regions of the motor strip on the cortical surface representing particular parts of your body), and the discovery of cortical columns by Vernon Mountcastle in 1957 (where neighboring cortical cells responded to similar input).
2. y Cajal, R. Croonian Lecture: La fine structure des centres nerveux. Proceedings of the Royal Society of London 55, 444–468 (1894).
3. With exceptions such as Edgar Adrian in the 1940s and Gordon Shepherd in the 1970s proving the rule.
Lead image: Tatiana Stulbo / Shutterstock