When Karley Campbell arrived at the North Pole for the first time, last year, it was raining. Even though she’d become a scientist, her implicit notions about the top of the globe had coalesced in childhood. It would be a solid place, with terra firma enough for at least one workshop of elves. Instead, she and her colleagues found lots of open water. “It was a somber, somber moment,” she recalls.
Campbell is a marine botanist at the Arctic University of Norway and the lead scientist on a project investigating “the metabolism of sea ice.” This may sound a strange pursuit in what would appear a barren, almost-otherworldly abiotic landscape. But the polar ice holds consequential secrets of, and for, life.
Scientific projects to understand the dramatic effects of the derailing climate are underway across the globe. Researchers are tracking warmer temperatures and rising seas, increasing droughts and wildfires, animals and humans on the move. Outside the spotlight, though, are projects like Campbell’s and people like her, taking account of incredibly subtle changes that will have lasting and uncertain effects on ecosystems—in this case, the ocean’s food web, which stretches from unseen microbes to us.1
A single milliliter of sea ice can contain upward of 1 million living bacteria.
It takes a certain type of person to dedicate their career to studying life our eyes can’t see, in a place our bodies aren’t suited for. Perhaps an eternal optimist. Or, like Campbell, a self-described pessimist. Whose outlook brings a certain essential pragmatism to the field—and to the field work.
“Anything that can go wrong will go wrong,” Campbell says from her office in Tromsø, Norway, a town in the Arctic Circle where the sun had set in November for the long polar night. An experimental set up that works swimmingly in the lab is bound to break in -40-degree temperatures, she says. Campbell admits she picked a strange line of work: “I’m actually always cold.”
To do this research, scientists need an astounding quantity of gloves—Campbell herself always has at least a half-dozen pairs—pathogenic bacteria, and hired guns. But the risky work is essential if we are to have even a fleeting hope of understanding the microbes’ roles and importance in the rapidly changing ecosystem. And what their disappearance might mean.
In the vast, bleak, windswept surface of our planet’s polar seas, buried within drifting slabs of sea ice, life is indeed not only present, but multitudinous. Bacteria, archaea, fungi, algae, viruses. All making their way in the otherworldly environment of polar sea ice, swimming in brine pockets, gobbling up carbon, feeding the food web in the depths below. A single milliliter of sea ice can contain upward of 1 million living bacteria.
As the polar autumn arrives each year and temperatures drop, ice crystals on the sea surface multiply, coalesce, and create larger sheets of sea ice. These overwintering drifters speckle the surface, joining larger amalgams of floating ice that persist for multiple years, growing and shrinking with the seasons. Often covered with a drift of snow, these sea ice chunks can range from several inches to more than 15 feet thick.
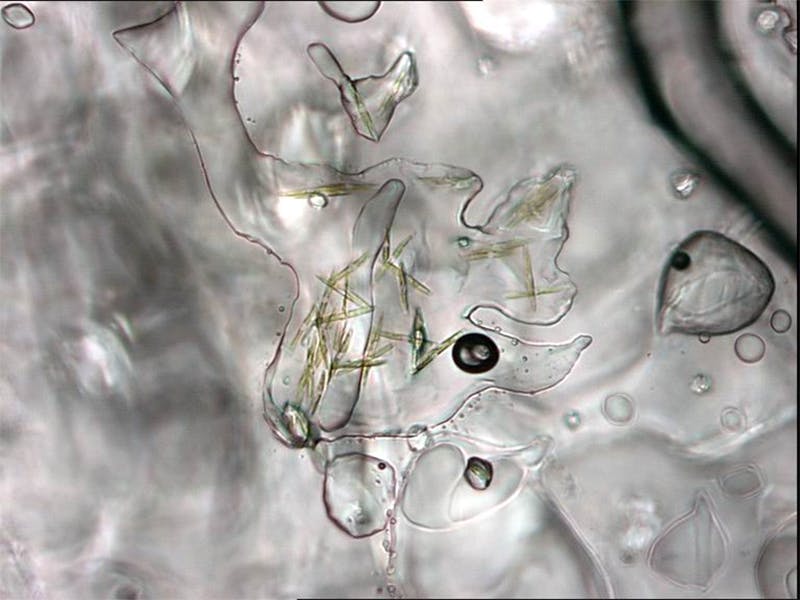
But far from a solid block of ice, they are worlds within themselves—gradients of light, temperature, and salinity, permeated with tiny pockets and channels of liquid brine. And it is primarily within these varied niches and along the slushy bottom that life has devised ways to persist well below freezing.
To avoid a certain death from ice crystals forming within their own bodies, microorganisms that thrive in this ice—which ranges from about 28 to -31 degrees Fahrenheit—have evolved a range of incredible adaptations. In one dramatic case of convergent evolution, life from multiple lines—bacteria to algae—have settled upon a sort of antifreeze compound.2 These large, goopy excreted sugars work a bit like a chemical version of an avalanche airbag, helping to keep open a livable pocket for themselves in the ice. (Even beyond shaping the ice itself, these microbe-generated substances might contribute to local weather, aerosolizing and spurring cloud formation.3)
Scientists have also uncovered how some of these microbes withstand the super salty brine. As the seawater crystalizes into ice, sodium is excluded, squeezing it into ever-increasing concentrations within the brine. With such spikes in salinity, researchers detected an increase in other helpful compounds that protect cells against too much salt.4
“You have to have somebody with you keeping watch for polar bears while you’re working.”
Rather than enter into a state of suspended animation or subdued hibernation, these microorganisms simply carry on doing much of the same things as when they are floating about in the relatively milder summer waters (often adapting to drastic changes in temperature and salinity across just days or hours5). Sea ice bacteria eat dissolved carbon and other organic material trapped with them in the brine, casting off other compounds in the process. This miniscule metabolic activity means they play a continuous role in cycling elements, such as carbon and nitrogen, making them a central, if underappreciated, force within the polar ecosystems through the harsh, starlit winters and dawn-soaked early spring.
The most profound contribution to these cycles happens in the cold, liminal world between ice and water, where an unexpected layer of algal life bursts forth. Dormant during the polar winters, these algae blossom when the sun begins to reappear. An algal cell will feast on carbon dioxide and nutrients expelled by bacteria or pushed up into the ice from the waters below. They shape the biogeochemical cycles in part by fixing carbon, which gets consumed by animals, giving some of it a chance to sink to the bottom of the sea where it could get sequestered for hundreds or thousands of years.
Although these algae cells make up a relatively small percentage of photosynthesizers in polar waters overall, their role is crucial. They bloom before open-water phytoplankton do, providing a reliable first course to zooplankton which become food for other locals higher up the food chain so they can begin their time-worn reproductive cycles, explains Campbell. And they are highly concentrated, which is of no small importance for marine grazers, who rely on them for copious buffets in a single location rather than having to skim about for diffuse fodder in the open seas. These ice-loving diatoms also tend to be especially rich in lipids, Campbell notes, an important foundation for the rest of the food chain.
Whenever Brian Lanoil and his colleagues embark on an expedition to collect microbes from the ice, they enlist a local trapper or hunter to come along. “You have to have somebody with you who is armed with a rifle, somebody keeping watch for polar bears while you’re working,” says Lanoil, a microbiologist at the University of Alberta.
The crews also must be vigilant about not sinking. On some trips to the deep Arctic, working just a few hundred miles from the North Pole, Lanoil reaches research locations by plane. “You trust the pilots to know what they’re doing—then you hop out of the plane and quickly drill a hole to make sure that the ice is thick enough to support the plane,” he says. “It’s wild stuff. It’s great.”
More common in Lanoil’s experience, however, are much tinier threats to his line of research: other microbes. Although the density of microbes in sea ice is surprisingly thick, it is nowhere near as vivacious as, for example, the soil outside of his university office in Edmonton. That means non-ice microbes picked up during the sampling, transport, or storage process pose a real threat to the delicate data he and others are trying to tease out.
They’ve been countering that eventuality by adding contaminants of their own. They paint the outside of an ice sample with E. coli, which is very content in the human gut but is most definitely not a sea ice resident. Equipped with a gene that makes it glow, this E. coli then acts as a stand-in for all other microbes that might have stowed away on samples. Then Lanoil and his colleagues douse the sample in bleach, which not only kills the exterior hitchhikers but also fries their DNA, preventing it from contaminating genetic screens of the sample later. Once the researchers can see the E. coli has been vanquished from the outside of the sample, they can carefully proceed to probe the inner regions of the ice sample to study the elusive endemic microbes to see what secrets of icy life they might be hiding.
As the Arctic warms and endures more rain days like Campbell experienced, its persisting multiyear ice is in danger of vanishing. Campbell notes with resigned certainty that this type of ice will be entirely gone from the Arctic before she retires. As another team of researchers put it, the Arctic Ocean will be “practically sea-ice free in September … before the year 2050.”6 (A certain workshop will soon need a flotilla.) It is the multiyear ice that offers far more gradients of temperature and salinity, providing more diverse habitats for microbes to inhabit, than the thinner seasonal ice that forms and vanishes with each tilt of the planet.
As ice habitats become more uniform first-year ice, “you end up losing a lot of niches for organisms, and so you end up getting much less diverse organisms,” Lanoil says. Which, in turn, he says, leads to a less efficient, less stable community. And these homogenizations mean the microbial communities are less resilient to further disturbances. Which Lanoil sees as a sort of feedback loop, as “changes to those environments just compound upon each other and get worse and worse.”
The ice acts as a sort of crucible for life that lives within it.
Lanoil and others have been looking to other reserves of ice on the planet to see what might be in store for marine ice microbes. Taking ice cores from Beringia, between Siberia and Yukon, he and colleagues have peered back in time to the changeover from the late glacial Pleistocene to the Holocene, across about 25,000 years ago to 15,000-11,000 years ago. Even with that transition, he says, “essentially the microbial communities didn’t change at all.” Similarly, looking at microbes across 11,000 to about 5,000 years ago, microbe profiles were also very stable. But the ice microbes from those two time periods look vastly different.7 Something switched. And they’re not sure why yet. It seems “these microorganisms are quite stable and able to adapt to a relatively minor change in the environment,” Lanoil says. A little warmer or cooler, and they can rev up or slow down their metabolism to adapt. “But if there’s a big enough change in the environment, then we get a dramatic shift,” he says. “There’s basically a tipping point we reach where the community can no longer adapt, and then we shift to a completely new one.”
We might be entering just such a transition now. And if so, “we’re going to see a dramatic shift in the microbial community with unknown consequences,” Lanoil says. With sea ice structure changing, “you’re losing entire communities of microorganisms that are really important for the functioning of Arctic ecosystems because the organisms that are living in and under the ice are really important for regulating the flux of food through the food chain,” Lanoil says. As a result, “you end up getting a very different big change in the ecosystem as a whole.”
But the implications are unclear. “We don’t know what that means, in terms of the ability of these organisms to actually carry out their ecosystem functions to do the important things that they do at the base of the food chain, that allow life to continue to do the things that it does,” Lanoil says. “We don’t really know what the consequences are—all we know is that it’s a very dramatic change,” he says. “We’re running an experiment where we don’t know what’s going to happen as a result, and we all pay the consequences of that.”
We might not see these shifts coming. For example, the microscopic algae that live and die beneath the ice are hidden from the peering view of satellites that track other ocean photosynthesizers. “But those of us who go up there and core the ice and sample it—or put divers or rovers or other autonomous vehicles to travel under the ice—we can witness and document the massive blooms,” says Jody Deming, a professor of biological oceanography at the University of Washington.
Deming has been venturing north, often under the cover of polar nights, to search for microscopic life since the 1970s. Once an undergraduate at Smith College studying botany on a piano scholarship, Deming is now in her 70s, with cheeks ruddied by years living among polar wind gusts. She jokes that a moment of frustration in thick gloves on her first Arctic research expedition gave her such severe frostbite it ended her concert piano career.
Since then, she’s been a leader in the field, making discoveries about these unexpected organisms, their unusual lives, and hidden importance. “For the Arctic ecosystem itself, that amount of under-ice blooming that’s going on is critical to the ecosystem,” Deming says. Not only that, but the ice acts as a sort of crucible for life that lives within it—that then eventually gets released back into the sea below. “The ice helps determine the nature of the microbial ecosystem and polar waters,” Deming says. “And that, of course, translates to all the biogeochemical cycles, and gas production, and climate impacts.”
After decades on the ice, she has seen the changes firsthand. The seasonal rhythms of the larger animals’ ancient patterns, built by microbes, are faltering, Deming says. “What they’ve adapted to forever, it’s now changing.”
Katherine Harmon Courage is the deputy editor at Nautilus.
Lead image: Jurjanephoto / Shutterstock
References
1. Campbell, K., et al. Monitoring a changing Arctic: Recent advancements in the study of sea ice microbial communities. Ambio 51, 318-332 (2022).
2. Ewert, M. & Deming, J.W. Sea ice microorganisms: Environmental constraints and extracellular responses. Biology (Basel) 2, 603-628 (2013).
3. Decho, A.W. & Gutierrez, T. Microbial extracellular polymeric substances (EPSs) in ocean systems. Frontiers in Microbiology 8, 922 (2017).
4. Firth, E., Carpenter, S.D., Sørensen, H.L., Collins, R.E., & Deming, J.W. Bacterial use of choline to tolerate salinity shifts in sea-ice brines. Elementa 4 (2016).
5. Firth, E., Carpenter, S.D., & Deming, J.W. Compatible solutes: Explaining how arctic microbes survive extreme salinity fluctuations, with relevance to the habitation of icy moons. Astrobiology Science Conference (2015).
6. Notz, D. & SIMIP Community. Arctic sea ice in CMIP6. Geophysical Research Letters 47 (2020).
7. Saidi-Mehrabad, A., et al. Permafrost microbial community structure changes across the pleistocene-holocene boundary. Frontiers in Environmental Science 8 (2020).