When Galileo Galilei first pointed a small telescope at the heavens in 1609, he began a revolution in astronomy. Today huge telescopes and radio dishes tell us about the universe. But they cannot directly probe those invisible sharply curved regions of spacetime called black holes or find those so far undiscovered invisible tunnels through spacetime called wormholes, theorized to offer instantaneous cosmic transport and possibly time travel.
Now, though, we have discovered gravitational waves, undulations in spacetime that can directly reveal black holes and wormholes by how they ripple the fabric of the universe. Rainer Weiss of MIT, who with Barry Barish and Kip Thorne of Caltech shared the 2017 Nobel Prize for the discovery, tells Nautilus that astronomical research in the wake of the initial observation “has produced so much science it’s unbelievable.”
Einstein predicted gravitational waves in 1916 after developing general relativity, his theory of gravitation that does not treat gravitation as a force, but as arising from the curvature of spacetime. He showed that an accelerating mass would generate gravitational waves moving at the speed of light, analogous to but different from the electromagnetic waves produced by accelerating electric charges. Einstein himself was unsure if they would ever be discovered, but he fully understood their importance as potentially giving direct experimental evidence of his view of spacetime as the underlying cosmic fabric.
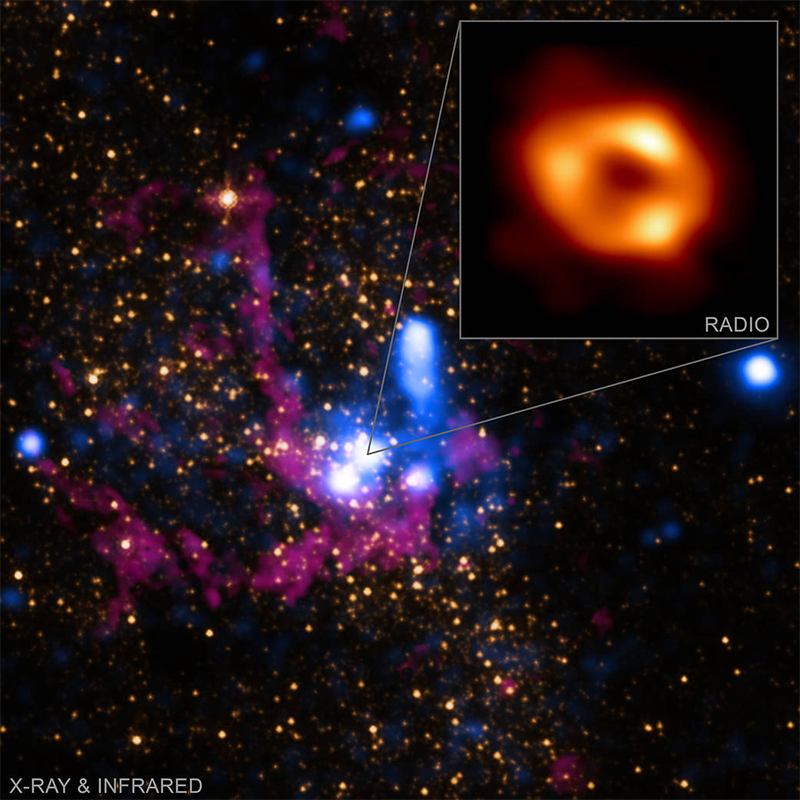
Einstein wondered about the discovery of gravitational waves because they are exceedingly weak, too weak to detect unless they come from massive bodies in rapid motion. Fortunately, these criteria are met by black holes when they collide. Gravitational waves were finally detected on Sept. 14, 2015, by two separate LIGO (Laser Interferometer Gravitational-Wave Observatory) installations in the United States.1 Each consists of two legs, both 4 kilometers long, that form an L-shape. Laser beams bouncing between mirrors traverse the legs and meet at the corner of the L, where their interference pattern responds to the tiny spatial changes as a gravitational wave sweeps by.
This first observation came from two black holes, 36 and 29 times as massive as our sun, when their mutual gravitational attraction sent them spiraling into each other, reaching half the speed of light just before collision. LIGO detected the resulting transient gravitational wave as a signal that accurately matched the prediction from general relativity. This direct evidence for gravitational waves also showed for the first time that two black holes can merge; in this case, into a black hole of 62 solar masses.
LIGO displayed remarkable sensitivity in registering this event, responding to distortions in space that changed the 4 kilometer distance between mirrors by less than the diameter of a proton. This feat jump-started an astronomical program at LIGO under the auspices of Caltech and MIT and in collaboration with the Virgo gravitational wave observatory in Italy, with the KAGRA observatory in Japan joining in 2020. By 2021 the consortium had observed 90 gravitational wave events. Centuries of electromagnetic wave astronomy have produced catalogs of stars and galaxies that classify them by their fundamental properties. Now gravitational wave astronomy is doing the same for black holes and their cousins, neutron stars, the dense objects that can form on the way to the formation of a black hole.
Most events observed so far represent mergers between two black holes over a range of masses. According to relativity, a black hole is defined by just three basic properties. Every black hole has mass, although a given black hole may or may not also spin in space or carry an electric charge. Accordingly, astrophysicists have created the first taxonomy of black holes by mass. Its three categories were determined indirectly before the advent of gravitational wave astronomy by observing the gravitational pulls of black holes on nearby stars or on dust and gas.
The first category is stellar mass black holes arising from the normal evolution of stars more massive than our sun. Like all stars, their nuclear fuel keeps them stable as it generates outward pressure against gravity’s inward pull. But when the fuel is gone, gravity rules, condensing the stellar material into a core. If the core contains one to three solar masses, compression continues until the protons and electrons in atoms merge into neutrons, producing a neutron star only 10-20 kilometers across. If the core exceeds three solar masses, compression never stops. It causes a stupendous supernova explosion, then squeezes the remaining core into an infinitesimal volume. This is a stellar black hole, containing up to 100 solar masses depending on the properties of the initial star.
Astronomy since the first observation of gravitational waves “has produced so much science it’s unbelievable.”
The second category comprises supermassive black holes with 100,000 to billions of solar masses, thought to lie at the centers of most galaxies. The supermassive black hole in our own Milky Way has the mass of 4 million suns (it and another supermassive black hole in the galaxy Messier 87 have just been “photographed” by a team of telescopes, showing the glow the black holes produce in the surrounding gas). In some extremely distant and therefore comparatively young galaxies, supermassive black holes appear as quasars, called “quasi-stellar radio sources” when first seen in 1962. Now we know these are supermassive black holes pulling in and heating surrounding matter until its glow can be seen for billions of light years. The central position and prevalence of supermassive black holes in galaxies, especially young ones, suggests they are integral to galactic birth and development. This remains to be explored along with the process that makes them so massive.
The vast difference in mass between stellar black holes and supermassive black holes has led to searches for black holes with masses between 100 and 100,000 solar masses. There is some indirect evidence that these exist, defining a third category of intermediate mass black holes, but few have been found and their formative process has been unknown.
The gravitational wave outcomes so far probe or have implications for all three categories. Many of the discoveries come from the latest results published in 2021. Of 35 gravitational events recorded, 32 represent mergers of pairs of stellar mass black holes of 10 to 90 solar masses, indicating the prevalence and wide range of this category. Notably, some mergers produced black holes larger than 100 solar masses. The most reliable of these observations, denoted GW190521, showed two colliding black holes of 85 and 66 solar masses yielding a remnant black hole of 142 solar masses, the first direct evidence of the formation of an intermediate mass black hole. Theorists propose that mergers of intermediate black holes within galaxies could contribute to the growth of supermassive black holes and galactic development.
Gravitational wave astronomy may also reveal a fourth, speculative category for black holes—primordial black holes, theorized to have formed soon after the Big Bang when fluctuations in the distribution of matter could have created ultra-dense regions. Possibly primordial black holes seeded supermassive black holes and so could be crucial to the formation of galaxies and cosmic structure. This alone would motivate the search for them, but there is another reason. If any primordial black holes have survived until today, they could answer the biggest open question in physics and astrophysics: What is the nature of dark matter, which cannot be observed by conventional astronomy? Until we understand this unknown substance that makes up 85 percent of all matter, we have an incomplete view of the universe. But so far no one has found appropriate new kinds of elementary particles that explain the existence of dark matter. Primordial black holes are possible candidates.
One initial LIGO/Virgo search for primordial black holes sought black holes of one solar mass or less, a tiny size that some theories suggest could have formed in the early universe. None were found, but two recent papers propose that other gravitational wave data may already show primordial black holes. One paper noted that under certain theoretical assumptions, the black holes in merger event GW190521 could have a primordial rather than a stellar origin.2 From this, the second paper suggested that statistical analysis of LIGO/Virgo data could reveal that primordial black holes already contribute to gravitational wave observations.3 The authors concede that truly confirming this “tantalizing possibility” would need better theories and finally observational verification. Finding primordial black holes would be a major triumph for gravitational wave astronomy and a breakthrough in cosmology, even more so if they are linked to dark matter.
Apart from the new knowledge of black holes, two of the 35 gravitational wave observations reported in 2021 represent a never-before seen cosmic event, a black hole-neutron star merger. Earlier, in 2017, LIGO/Virgo had made the first observation of two colliding neutron stars. Both kinds of interactions will reveal more about these dense stars, but according to Weiss“the thing that caused the biggest stir is the neutron star collision.” This was the first event ever to be tracked by both gravitational wave and electromagnetic wave methods, known as “multi-messenger” astronomy, which gave a rich set of results.
For instance, the LIGO/Virgo data alone could only place the collision within the constellation Hydra, but when electromagnetic wave observatories around the world examined the light emanating from the resulting explosion, the event was placed more precisely near the galaxy called NGC 4993. The dual analysis also showed that the collision produced chemical elements heavier than iron, such as gold and platinum, giving further understanding of the process whereby stars make the heavy elements. Third, the near-simultaneous arrival of gravitational waves and electromagnetic waves from the same source 130 million light-years away definitively confirmed Einstein’s prediction that gravitational waves travel at the speed of light. Finally, multi-messenger analysis also provided a new way to measure the Hubble constant, the crucial parameter that describes how fast the universe is expanding and appears in all cosmological theories.
Could the huge mass at the center of the Milky Way be the end of a wormhole rather than a black hole?
Multi-messenger astronomy offers other possibilities, such as probing the spinning neutron stars called pulsars. These can be located because they emit beams of electromagnetic wave radiation that arrive at regular intervals as they sweep across the Earth like a lighthouse beam. If the neutron star is asymmetric, it will also emit gravitational waves as it rotates, giving further clues to the star’s hyper-dense structure. In 2021 LIGO/Virgo used electromagnetic wave and gravitational wave methods to select and examine 18 pulsars.4 No gravitational waves were detected but a new observing run is planned with higher sensitivity.
Another especially exotic multi-messenger project would also need higher sensitivity. It is inspired by speculation that the huge mass at the center of the Milky Way is not a supermassive black hole but one end of a wormhole. Some researchers propose that ultra-precise electromagnetic wave measurements of stars orbiting the galactic center could differentiate a supermassive black hole from a wormhole; others believe that gravitational wave data could make the distinction. Theorist Aurélien Hees of the Paris Observatory, who worked with the stellar orbital measurements that found the 4 million solar masses at our galactic center, thinks that neither form of astronomy is yet sufficiently exact. But, he adds, next-generation electromagnetic wave telescopes and gravitational wave detectors might together explore this intriguing possibility.
In electromagnetic wave astronomy, “next-generation” means telescopes with ever-bigger mirrors or radio dishes to better gather dim light from the distant universe. In gravitational wave astronomy, “next-generation” means ever-longer detectors. The spatial change in length registered by a laser interferometer is proportional to the distance between the interferometer’s mirrors: The greater the distance, the more sensitive the detector (many other factors affect sensitivity as well). Also, like any wave, a gravitational wave has a frequency, which is smaller the larger the originating mass and depends on how it moves. A detector with a broad frequency range can register more varied types of gravitational wave events.
These principles guide the planning of enhanced gravitational wave observatories such as the Laser Interferometer Space Antenna (LISA) proposed by the European Space Agency.5 Three orbiting spacecraft carrying lasers and mirrors would form an ultra-sensitive triangular interferometer with legs 2.5 million kilometers long. Distant from Earthly sources of measurement noise, LISA could detect gravitational wave frequencies far below 1 Hz, allowing new studies of supermassive black holes. LISA would not be launched until 2037, but ground-based upgrades could come sooner. European scientists are proposing the Einstein Telescope, a triangular interferometer with three 10 kilometer-long arms, and U.S. scientists propose the Cosmic Explorer. It would follow the LIGO “L” design with arms 40 kilometers long, giving a nominal tenfold increase in sensitivity with other performance improvements.
Weiss is helping to design this larger device. It had long been his dream, he tells me, but the original National Science Foundation (NSF) funding for LIGO in the 1990s would only pay for 4-kilometer arms. The Cosmic Explorer would carry gravitational wave astronomy toward what Weiss considers the next big area for gravitational waves to illuminate, which personally enthralls him: cosmological questions about the early universe such as the existence of primordial black holes, their links to dark matter, and closer looks at how the universe expanded and how the stars and galaxies were made based on the gravitational waves these processes generate. New measurements would also continue to test Einstein’s theory to ever greater precision, but there Weiss doesn’t expect any surprises. Because general relativity has so far passed every experimental test, Weiss says he would still “put my money on Einstein.”
As new gravitational instruments are built, the result will be a group of gravitational wave observatories operating over different frequency ranges, much as conventional observatories examine electromagnetic waves of different frequencies from gamma rays to radio waves. That spectral breadth gives electromagnetic wave astronomy its wide window on the universe. Now seven years into the regime of gravitational wave astronomy, a wide gravitational window is opening to give a unique but complementary view of the cosmos. We can hardly imagine what wonders this dual vision will uncover.
Sidney Perkowitz is the Candler Professor of Physics Emeritus at Emory University. His latest books are Physics: A Very Short Introduction, Real Scientists Don’t Wear Ties, and, just released, Science Sketches: The Universe from Different Angles.
Lead image: Jurik Peter / Shutterstock
References
1. Abbott, B.P., et al.Observation of gravitational waves from a binary black hole merger. Physical Review Letters 116, 061102 (2016).
2. De Luca, V., Desjacques, V., Franciolini, G., Pani, P., & Riotto, A. GW190521 mass gap event and the primordial black hole scenario. Physical Review Letters 126, 051101 (2021).
3. Franciolini, G., et al. Searching for a subpopulation of primordial black holes in LIGO/Virgo gravitational-wave data. arXiv 2105.03349v3 (2022).
4. The LIGO Scientific Collaboration, et al. Narrowband searches for continuous and long-duration transient gravitational waves from known pulsars in the LIGO-Virgo third observing run. arXiv 2112.10990 (2021).
5. Cho, A. Giant detectors could hear murmurs from across universe. Science 371, 1089-1091 (2021).