Celebrated playwright Terrence McNally passed away on March 24. As told to The New York Times by his husband Tom Kirdahy, the cause of death was “complications of the coronavirus.” McNally, 81, died on the battlefield of his body. He was a lung cancer survivor. Treatment had left him with COPD, a condition caused by accumulation of damage to delicate gas exchange tissues in the lungs. When McNally accepted a Tony Lifetime Achievement Award in 2019, he appeared on stage assisted by breathing tubes and darkly commented that the award came “not a moment too soon.” He died while infected with SARS-CoV-2. But what is it that killed him?
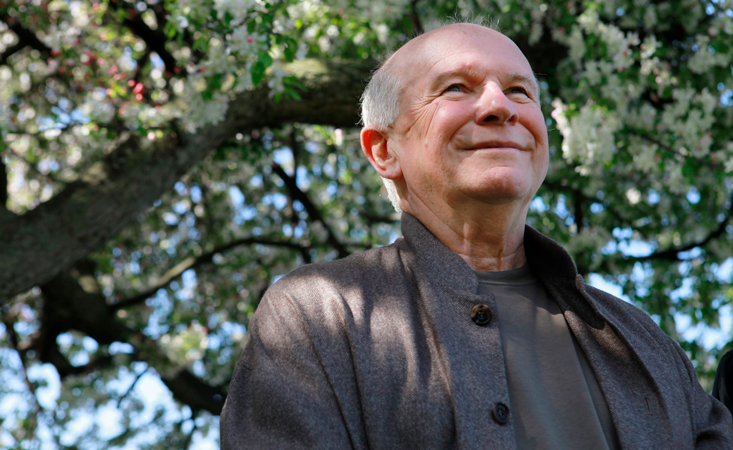
Understanding the role that the infection played in McNally’s death requires us to examine the epidemic as a whole. Two common measures of a pandemic are R0, R-naught,1 and CFR, the case fatality rate.2 The former measures transmission of the virus—how many new infections are, on average, generated by an infected individual; the latter how many infected will die of the virus. Both statistics require an accurate count of the absolute number of people who have the virus. But getting a solid estimate of that number has been hampered by the shortage of accurate testing kits,3 the lack of trained technicians to perform the tests,4 and tests that repeatedly give different results for the same individual when they’re finally carried out.5
Measuring R0, the ease of contagion, is no less difficult. Understanding the spread of the virus requires tracing all social interactions of infected individuals. This is possible at the start of epidemics where new cases originate from known cases, but it approaches impossible when there’s community spread—new cases coming from unknown sources. Both CFR and R0 are useful parameters that help us discuss an epidemic that has already unfolded—but in the midst of one that’s defined by a lack of testing capacity, these numbers can be mercurial. And, on top of that, while they convey the very real impact of the outbreak, they fail to explain why the virus is so dangerous.
To understand that, we have to look at the health of those afflicted. The numbers show that, across the board, COVID-related fatalities skew older. There are exceptions—as demonstrated by the deaths of frontline medical workers, children, and healthy adults—but reading through available obituaries of COVID-19 victims suggests many of those who succumb do so because their bodies are weakened by decades of chronic illnesses.
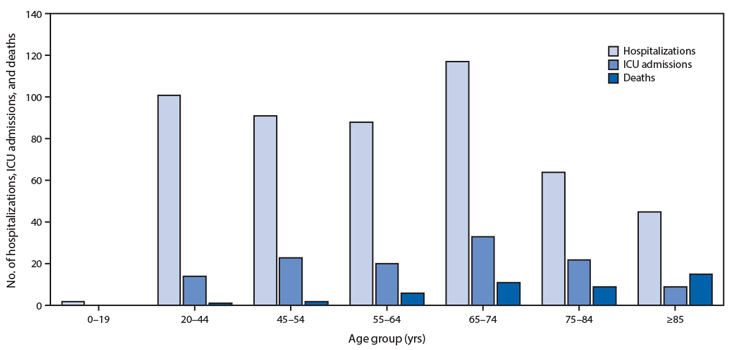
The theory that this respiratory virus preys on the weakened is further bolstered by data from the Spanish and Italian health ministries,6,7 which show that the majority of the dead in both countries had multiple comorbidities, the medical term for antecedent diseases. In fact, only 1.4 percent of tallied fatalities in either country occurred in individuals with no history of life-threatening medical conditions such as ischemic heart disease, stroke, COPD, or chronic renal failure. In this context, it’s hard to read the SARS-CoV-2 infection as the cause of death. The accelerator of death? Absolutely. But the chronic health conditions in reported fatalities indicate that many patients were already seriously ill before the virus arrived.
Before breathing a sigh of relief, let’s take a moment to consider the data. As shown in the chart below, reproduced from Aging and the Epidemiology of Multimorbidity,8 almost 25 percent of people have at least one chronic disease by the age of 35. By the time they reach the age of 60, it’s almost 50 percent. By the time they reach 75, it’s 75 percent. These illnesses accumulate with age, and a significant percentage of older individuals battle multiple serious diseases.
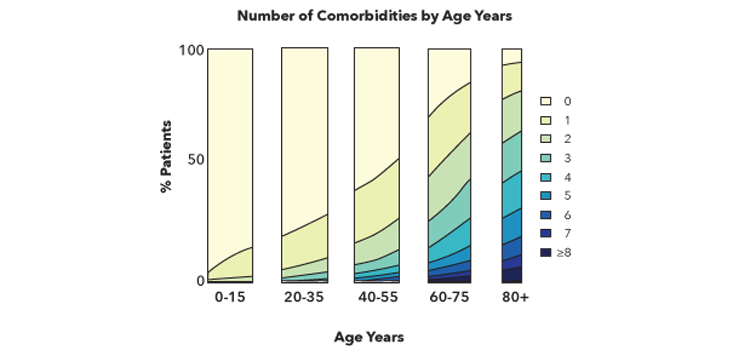
For those with multiple chronic conditions, irrespective of their age, infection with SARS-CoV-2 is like tossing a lit match into a powder keg. But what exactly do these pathologies have in common that results in this fatal summation?
In healthy aging, individuals grow older without accumulating a burden of chronic diseases.9 On the other hand, those who undergo unhealthy aging gradually accumulate chronic conditions. These illnesses can result from diet, substance-abuse, smoking, environmental pollution—or they might simply be due to genetics. Whatever the source, a chronic condition is, by definition, marked by cellular dysfunction. Many common comorbidities, including heart disease, cancer, chronic bronchitis, or emphysema, often include a dangerously prolonged inflammatory response. Inflammation is a condition that results from the immune system’s aggressive defense against injury or foreign invaders, and can be activated by disease.
Non-functional or dysfunctional cells are as much of a threat to the body as a pathogen. Evolution likes to keep it simple, and so the immune system does double duty—it addresses both. Immune cells—both adaptive and innate—program damaged cells to die; flag and remove dead cells; and sense, integrate, and address molecular distress signals. Under normal conditions, this spurt of activity quiets down, the organ regenerates, and individuals continue on with their lives. But in chronic illnesses, cells get sick and die constantly.
When cellular damage is registered, the body responds with tactical immunological force. On the front line of defense are surveillance cells, which release small proteins, cytokines, upon encountering a threat. Cytokines recruit white blood cells, the next stage of the immune response. They also act in concert with other inflammatory molecules to alter physiology and permeabilize blood vessels, changes that support a more effective threat response.
This nonspecific response, sustained for years by one or more chronic medical conditions, turns much more deadly in the presence of a pathogen. The external threat recruits granulocytes, a subset of the circulating immune cells. Upon arrival, they disgorge the caustic contents of the granules they’re named for. This payload largely consists of small proteins that act as a nonspecific defense mechanism against pathogens—lysozyme, antimicrobial peptides, superoxides, and acids. Granulocytes are effective at stopping the infection, but the corrosive contents of their granules can cause widespread structural damage in the organs that they target.
One one hand, increased permeability of blood vessels helps immune cells reach the site of infection. On the other hand, it can lead to even more severe infections because there’s no way to selectively allow passage of immune cells while screening pathogens. The entry of pathogens into sterile areas of the body, such as the blood, establishes a feedback loop where the nonspecific action of the immune system exacerbates the vulnerability of affected organ systems. When the circumstances are sufficiently dire, measured by the accumulation of specific chemical signals in the blood, the brain may initiate a last-ditch response: fever.
According to the report by the Italian Health Ministry, fever and labored breathing were the most commonly reported symptoms in deceased Italian COVID-19 patients.6 Labored breathing, given the fact that the virus attacks the lungs and the fact that more than half the patients had cardiovascular comorbidities, makes sense. But why fever?
Scientists aren’t completely certain if fever helps or harms. The rise in core body temperature may assist the body in fighting off an infection.10 Function of the innate immune system increases with temperature,11 and bacterial reproduction is inhibited.12 However, drawn out fevers can damage the host of their own accord.
The increased temperature setpoint of the febrile response accelerates the damage already wrought by an overactive innate immune system. With rising temperatures, widespread tissue damage accelerates: Cell-membranes disrupt, mitochondria cease functioning, DNA mutates, cell-signals are lost in the noise. Basement membranes, the structures that block free passage of microbes between the gut and the blood, already eroded from years of chronic inflammation, permeabilize further. In the most extreme cases, this can lead to endotoxemia—a condition where gut flora is free to enter the body. This wave of pathogens, happy to replicate in a warm, wet body whose immune system can no longer stem the tide, simply overwhelms. There aren’t enough resources to go around; there are not enough healthy cells to serve as a reservoir for repopulating organs that consist of more damaged cells than functional ones.
When a patient has a history of waging an intense, inflammatory battle against failing cellular machinery in multiple organ systems, they’re already near the end. Adding a viral infection to the mix can lead to a feedback loop where heightened cytokine chatter among cells further confuses immune responders, which promotes an even greater nonspecific reaction. The collateral damage of this cascade can allow entry of gut bacteria into the body. Eventually, the attempt to stave off the inevitable becomes too much.
The bottom line is that the impact of COVID-19 will be disproportionately absorbed by the chronically inflamed and the immunocompromised. Most United States citizens over the age of 65 have at least one major inflammatory condition, and their high level of risk warrants commensurate precautions. Perhaps the most concrete conclusion that can be made is about individual and societal decisions that will affect outcomes in future pandemics. Making health a priority, especially in the context of minimizing baseline inflammation, will serve us in years and generations to come. We’ve heard it all before—but the lethality of SARS-CoV-2 makes it painstakingly apparent.
Anastasia Bendebury studied the role of electricity in bacterial communication during her Ph.D. at Columbia University. She’s also studied infectious disease, aging, and reproductive medicine.
Michael Shilo DeLay studied nanoscale mechanics of cell signaling during his Ph.D. at Columbia University, after working on cell-signaling for nearly a decade at Yale, Ohio State, and UCSF. They’re the creators of Demystifying Science, a company devoted to providing clear, mechanistic explanations for natural phenomena.
References
1. Delamater, P.L., Street, E.J., Leslie, T.F., Yang, Y.T., & Jacobsen, K.H. Complexity of the basic reproduction number (R0). Emerging Infectious Diseases 25, 1-4 (2019).
2. Ejima, K., Omori, R., Cowling, B.J., Aihara, K., & Nishiura, H. The time required to estimate the case fatality ratio of influenza using only the tip of an iceberg: Joint estimation of the virulence and the transmission potential. Computational and Mathematical Methods in Medicine 42, 978901 (2012).
3. Baird, R.P. Why widespread coronavirus testing isn’t coming anytime soon. The New Yorker (2020).
4. Wetsman, N. Coronavirus testing shouldn’t be this complicated. The Verge (2020).
5. Krieger, L.M. Coronavrius false test results: With the push to screen come questions of accuracy. Mercurynews.com (2020).
6. COVID-19 Surveillance Group. Characteristics of COVID-19 patients dying in Italy. Report based on available data on March 20th, 2020. https://www.epicentro.iss.it/coronavirus/bollettino/Report-COVID-2019_20_marzo_eng.pdf
7. Spanish Health Ministry. Scientific and technical information on COVID-19 (2020). https://www.mscbs.gob.es/profesionales/saludPublica/ccayes/alertasActual/nCov-China/documentos/20200326_ITCoronavirus.pdf
8. Divo, M.J., Martinez, C.H., & Mannino, D.M. Ageing and the epidemiology of multimorbidity. European Respiratory Journal 44, 1055-1068 (2016).
9. Castelo-Branco, C. & Soveral, I. The immune system and aging: A review. Gynecological Endocrinology 30, 16-22 (2014).
10. Rantala, S., Vuopio-Varkila, J., Viento, R., Huhtala, H., & Syrjänen. Predictors of mortality in beta-hemolytic streptococcal bacteremia: A population-based study. Journal of Infection 58, 266-272 (2009).
11. Rice, P., et al. Febrile-range hyperthermia augments neutrophil accumulation and enhances lung injury in experimental gram-negative bacterial pneumonia. The Journal of Immunology 174, 3676-3685 (2005).
12. Small, P.M., Täuber, M.G., Hackbarth, C.J., & Sande, M.A. Influence of body temperature on bacteria growth rates in experimental pneumococcal meningitis in rabbits. Infection and Immunity 52, 484-487 (1986).
Lead image: Nyord / Shutterstock