In December 2015, particle physicists were buzzing with excitement: The Standard Model—which has dominated physics for 40 years and defines the basic constituents of matter and how they interact—had a new challenger. At the Large Hadron Collider in Switzerland, physicists announced evidence of what appeared to be a new particle. Known colloquially as the “diphoton bump,” the new particle promised to upend the Standard Model, which doesn’t predict its existence. It also opened the door to the possibility of solving long-unanswered puzzles, including the nature of dark matter.
About a month later, another potential challenger emerged. A group of nuclear physicists out of the Hungarian Academy of Sciences’ Institute for Nuclear Research published a paper on an anomaly detected in the decay of beryllium-8. They proposed that the irregularity could be the signature of a “dark photon,” one of the force carriers thought to dictate the action of dark matter.
On the surface, neither of these experiments might seem more viable than the other. Yet the response to each could not have been more different. While hundreds of theoretical papers were published on the “diphoton bump,” almost none followed the Hungarian paper.
For almost eight months, the Hungarian paper remained in obscurity, until a group from the University of California-Irvine posted their own theory about the anomaly detected in the decaying beryllium-8. Though their analysis didn’t support the suggestion that the anomaly was a dark photon, they thought it would fit the behavior of a new type of light, neutral boson—the class of particle that includes both photons and force-conveying particles like the Higgs boson. Yet unlike other force-conveying particles, their proposed particle interacted only with neutrons, and not with protons and electrons. Appropriately, they called it a “protophobic X boson.”
As the UC-Irvine team gave the Hungarian experiment visibility, the community began to respond. Yet unlike the physicists of the Large Hadron Collider experiment, who were met with enthusiasm, the Hungarian scientists mostly faced skepticism.
New ideas are often judged for how far they lie outside of the systems that scaffold our understanding of the world.
What caused such a dichotomy? Though many factors have since been cited, including the reputation of the Hungarian scientists, it seems the true difference between these two experiments comes down to something subtler: Expectation, and divergence from those expectations.
In the sciences, new ideas are often judged for how far they lie outside of the systems that scaffold our understanding of the world— systems that are not only scientific, but also social. But when it comes to solving our most persistent mysteries in physics, like the composition of dark matter—which has so far resisted all attempt at elucidation by traditional physics—claims from outside this paradigm may be vital.
The first strike against the beryllium-8 experiments is a scientific one: The model of the universe that it requires also needs physics well outside what is predicted by the Standard Model.
Though the team from UC-Irvine found that the Hungarians’ data did not contradict any existing experiments, the model they proposed to explain the new particle needed to be an intricate one. After all, the scientists had to explain why this new particle would not have shown up in years of previous experiments. They suggest a particle that interacts with neutrons, but not protons, and which experiences a hitherto unknown force with a range about 12 times the size of a proton. It is this model with which many scientist take issue.
“The question is, why would nature choose such a complicated model just to explain this phenomenon?” said Rouven Essig, a professor of physics at Stony Brook University’s C.N. Yang Institute for Theoretical Physics.
“We’ve got such a beautiful, nicely consistent theory in the Standard Model,” Essig said. “If something comes along that doesn’t fit any of that, and perhaps requires a unique, intricate new model to make it fit with anything, then that’s when it makes us very skeptical.”
There are other scientific aspects of the beryllium-8 experiment that can, and have, been raised as concerns. The Hungarian experimenters work mostly in nuclear physics, not particle physics; their detection came on a single small device, one many magnitudes less sensitive than the two massive, top-of-the-line detectors—ATLAS and CMS—which double-check every discovery in the Large Hadron Collider.
The group also published two previous papers with similar claims of new particles, including a 2008 claim of a potential 12-MeV particle, and a 2012 claim of a 13.45-MeV particle. Indeed, the Atomki researchers seem to have a penchant for publishing only papers with anomalies from their beryllium-8 experiments, what some see as potential confirmation bias.
These concerns play into the second strike against the beryllium-8 experiment. However, this second strike is more sociological than scientific.
“Where things get a little less defensible is that some of [the community’s skepticism] has to do with the fact that this is not a place where we thought physics was going to show up,” explains Tim Tait, a theoretical physicist from UC-Irvine, and a member of the group that brought the Hungarian experiment out from obscurity with their own theory. He says that this assessment applies both to the laboratory the proposed particle came out of, and the category it falls into—being a lighter-mass particle, physicists would expect to have observed it already in previous experiments. Neither is what the community would have anticipated as the source of new physics.
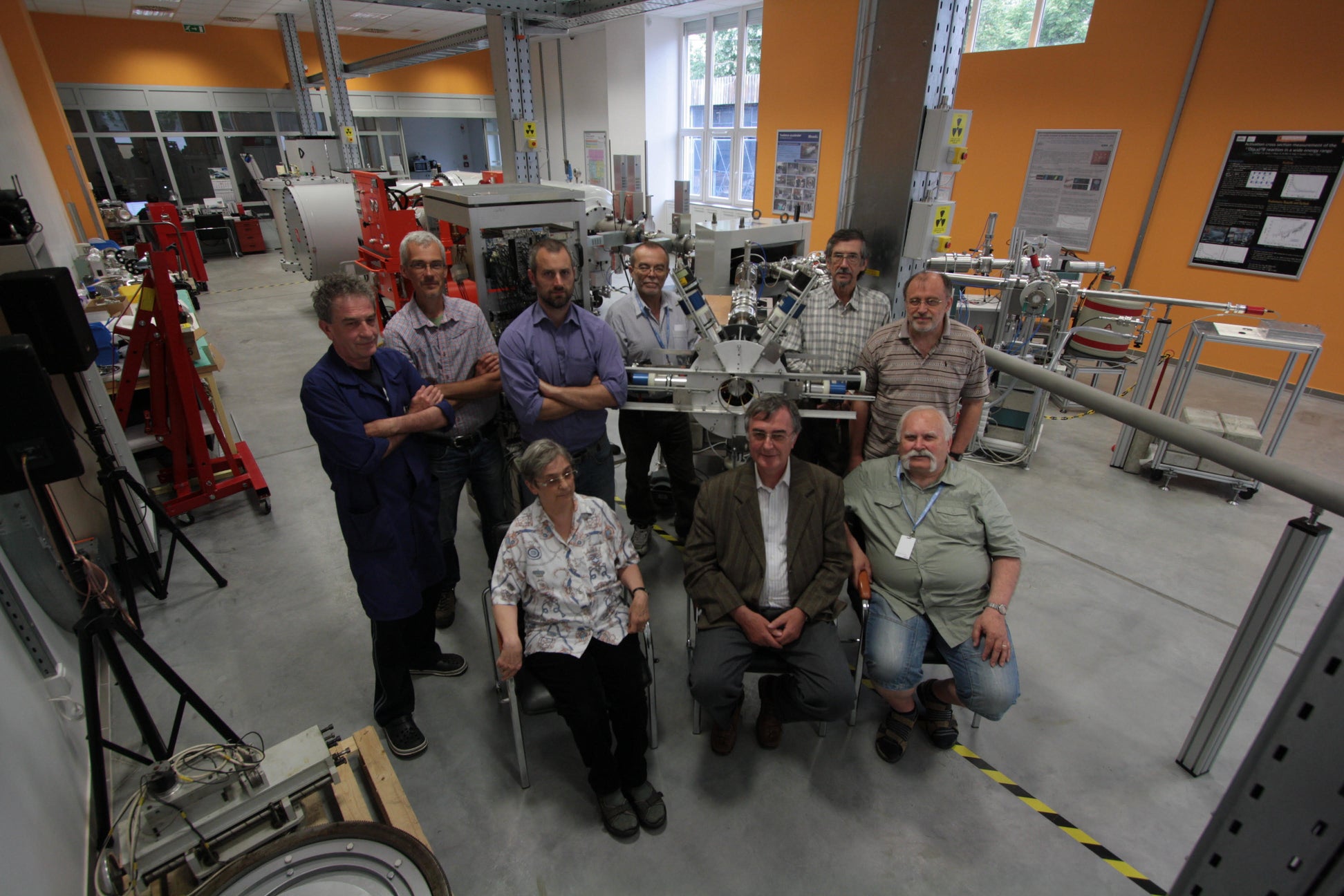
Tait says that when translated from a personal to a community level, these expectations are what lead to certain types of experiments, or certain places, being perceived as inherently more trustworthy than others. The diphoton bump, he says, is an almost textbook example of this.
The fluctuation that later became known as the diphoton bump was measured after a renovation, one that allowed the Large Hadron Collider to run at higher energies than before—the sort of energies that physicists expect to produce new particles. A particle that sat well outside of the Standard Model apparently did not seem so dubious in a program run by well-known physicists, in the very same facility where the Higgs Boson had been discovered not too long before—in short, a place where new particles were expected to appear.
“Lots of people came up with reasons that the diphoton excess was exciting, even though they knew it wasn’t statistically significant,” Tait said. “I think a lot of it comes down to the fact that these experiments at the LHC are very familiar territory. We trust these guys, we think they know what they’re doing, and their detectors are very sophisticated.”
It makes sense that scientists would rely on heuristics, like reputation and the standard theories of the field, as provisional rules of thumb. In judging a new experiment, scientists have only the experimenter and her experiment to go on. And while experimental data is often viewed as the ultimate arbiter between theory and fact, experiments themselves are not infallible. As sociologist of science Harry Collins laid out in his theory of the “experimenter’s regress,” facts can only be produced by good instruments, but good instruments are only considered such if they produce facts.
A long-standing reputation for doing work that has been replicated, or a set of data that easily slides into what is already understood, provides extra reassurance that this regress (or other potential distortions) played no part in a new idea.
Yet clearly these heuristics are imperfect. Exhibit A is the diphoton excess: Even with its impeccable pedigree, the new particle turned out to be an anomaly, and all the attention it received was for naught. Meanwhile, despite general skepticism around the unknown team out of Hungary, no significant objections have yet been raised about the team’s experimental results.
Take another example, this time from cosmology. In March 2014, a team working on the BICEP2 telescope, led by a researcher from Harvard–Smithsonian Center for Astrophysics, announced that they had detected polarization in the cosmic microwave background. This polarization would signal the existence of gravitational waves created by the expansion of the universe. It was a discovery that had been long anticipated; it was predicted by the inflationary theory of the universe, which like the Standard Model, guides cosmologists’ understanding of their field, but is never observed. Thus, the announcement was received enthusiastically at first. It fit with what was expected and who was expected to find it, and was widely accepted at face value. Over the course of the next year, however, it was proven that the signal could be attributed to cosmic dust.
In the face of these dilemmas, the question is: Are there more effective heuristics for judging new results?
A case could certainly be made for “no.” After all, it’s difficult to conceive of other criteria to judge new physics on face value. Being more receptive to outsiders and theories, and following up diligently on these claims, would flood the field and take up valuable time needed to investigate the most promising leads. The above incidents are also natural failures of the sort that we should expect out of such a huge and unfathomable field of science.
“We often judge new physics models by how well they can explain phenomena and how simple they are, but Nature may or may not care about our taste.”
What’s more, those who study the history of science see it as unlikely that such a drastic change in culture will occur.
“The way in which a community behaves is constructed over a long social progress, made by power structures, years of training, reward systems, rules of competition and collaboration between and within different groups,” says Roberto Lalli, research scholar at the Max Planck Institute for the History of Science. He says that history has shown that subcultures within physics—such as theoretical or particle physics—are relatively stable, and that it’s likely that places like CERN and ideas within the paradigm will continue to be considered the most plausible.
“This attitude is not only due to authority bias, but also has to do with first-hand knowledge of the internal reviewing systems within experimental groups,” Lalli said. “This creates a system of trust, which will not change in a sudden way.” Social pressures, like the continual fight for funding and university positions, also make communities more unwilling to accept those from outside the mainstream.
But a case still can, and should, be made for seeking new standards for the system. A sterling reputation can be hard to come by in a digital world, where obtaining visibility can be like shouting over a million voices, and the difficulty of the academic job market has spread talent widely beyond the most well-known institutions. Additionally, outsider ideas can help break the echo chamber that comes of only speaking to those within a relatively closed community.
Indeed, one of the most groundbreaking physicists in history could be framed as an outsider from the onset.
Albert Einstein was a low-level employee at the Swiss Patent Office when he proposed his special theory of relativity and his photon hypothesis (which theorized that light consists of individual particles, or quanta) in 1905. Though social structures were vastly different within physics in the early 20th century, hindsight of Einstein’s progress suggests that current outsider ideas may simply require time to pass before they can be accepted.
“The way in which [Einstein’s ideas] became part of the new paradigmatic framework was not rapid,” says Lalli. “It took years and a lot of work, of reformulation of previous knowledge, to fully understand the radical physical implications contained in the new theories.” For example, Einstein’s photon hypothesis was nearly universally rejected at first, and was only accepted in the late 1920s after the discovery of the Compton effect.
For the same sort of thing to happen today, Lalli says it “might not necessarily involve a change in culture. Rather, new ideas coming from unexpected places would gradually be included in the mainstream culture.”
As for the trusted standard of the Standard Model, physicists readily acknowledge that there’s much outside the current theories that we likely do not know. As Essig put it: “We often judge new physics models by how well they can explain phenomena and how simple they are, but Nature may or may not care about our taste.”
This also wouldn’t be the first time that nature was revealed to be much more complex than humans expected. In the beginning of the 20th century, when scientists first began experimenting on the scale of the atom, they saw particles behaving with zero regard for the laws of physics they understood. The birth of quantum physics required scientists in the field to rethink everything they knew about the laws of the universe—in essence, to throw out their textbooks.
A new culture of particle physics as a field of small experiments from outsider physicists, as well as huge ones from trusted groups, would not take such a dramatic transformation. It would perhaps require just the writing of a few new chapters.
Photo Credit: Caleb Perkins / EyeEm