In less than five milliseconds, a Hydromantes salamander can launch its tongue—including the muscles, cartilage, and part of its skeleton—out of its mouth to snag a hapless insect mid-flight. Among amphibians, it is the quick draw champ. Frogs and chameleons are comparative slowpokes when it comes to their ballistic anatomies. “I’ve spent maybe 50 years studying the evolution of tongues in salamanders,” says David Wake, an evolutionary biologist at the University of California, Berkeley, “this is a particularly interesting case because salamanders, who don’t do anything fast, have the fastest vertebrate movement I’m aware of.” Within their lineage, evolution found a better way to accomplish tongue-hunting. Their seemingly unique adaptation appears to have evolved independently in three other unrelated salamander species. It is a case of convergent evolution—where different species separately developed similar biological adaptations when faced with the same environmental pressures. Salamanders are Wake’s go-to example when asked a decades-old question in evolutionary biology: If you could replay the “tape of life” would evolution repeat itself? In the salamanders, it appears it has: In other organisms, it may not have.
This question was famously posed by the late evolutionary biologist Stephen Jay Gould in his 1989 book, Wonderful Life: The Burgess Shale and the Nature of History, which was published at a time when people still listened to music recorded on cassette tapes.1 The book discussed fossils left behind by myriad strange animals that inhabited the Earth’s oceans about 520 million years ago during the Cambrian period, and were preserved in the Burgess Shale. Nearly all animals alive today can trace their lineages back to the creatures that lived in the Cambrian, but not every animal that lived in the Cambrian period has descendants that live today. Many Cambrian species have since died out because they weren’t fit enough to compete, or because they were in the wrong place at the wrong time during volcanic eruptions, asteroid impacts, or other extinction events.
Gould saw the incredible diversity of the Burgess animals and theorized that life today would have been different had history unfurled in another way. Random mutations and chance extinctions—events Gould called “historical contingencies”—would build on each other, he suggested, driving the evolution of life down one path or another. In Gould’s view, the existence of every animal, including humans, was a rare event that would have been unlikely to re-occur if the tape of life were rewound to the Cambrian period and played again. One of the paleontologists—Simon Conway Morris of Cambridge University—whose work on the Burgess fossils was heavily cited by Gould in his book, strongly disagrees with this viewpoint.
In Gould’s view, the existence of every animal, including humans, was a rare event that would have been unlikely to re-occur if the tape of life were rewound to the Cambrian period and played again.
Conway Morris believes that, over time, natural selection leads organisms to evolve a limited number of adaptations to the finite number of ecological niches on Earth. This causes unrelated organisms to gradually converge on similar body designs. “Organisms have to configure themselves to the realities of the physical, chemical, and also biological world,” he says. In Conway Morris’s view, these constraints make it all but inevitable that if the tape of life were replayed, evolution would eventually reproduce organisms similar to what we have today. If humans’ ape ancestors had not evolved big brains and the intelligence that goes with them, he believes that another branch of animals, such as dolphins or crows, might have, and filled the niche that we now occupy. Gould disagreed.
Both scholars recognized that convergence and contingency exist in evolution. Their debate instead revolved around how repeatable or unique key adaptations, like human intelligence, are. Meanwhile, other biologists have taken up the puzzle, and shown how convergence and contingency interact. Understanding the interplay of these two forces could reveal whether every living thing is the result of a several-billion-year-long chain of lucky chances, or whether we all—salamanders and humans alike—are as inevitable as death and taxes.
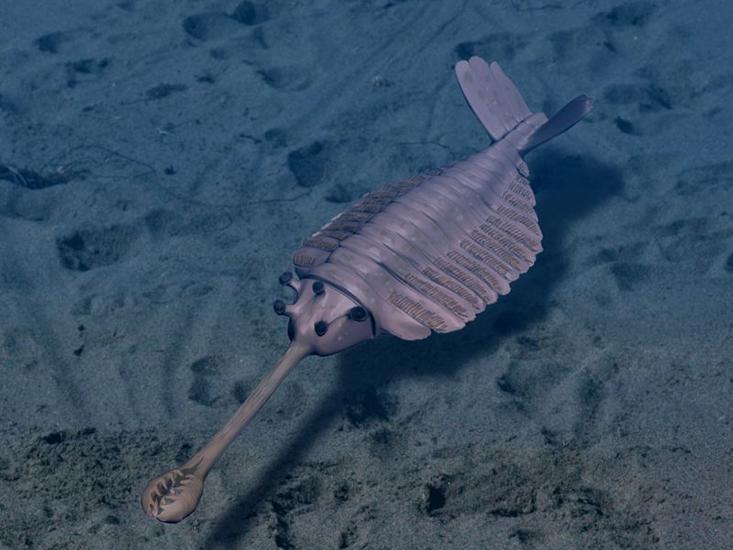
Rather than attempt to reconstruct history with fossils, Richard Lenski, an evolutionary biologist at Michigan State University, decided to watch convergence and contingency unfold in real time, in the controlled environment of his laboratory. In 1988, he separated a single population of Escherichia coli bacteria into 12 separate flasks containing liquid nutrients, and let them each evolve separately. Every few months for the past 26 years, he or one of his students has frozen a sample of the bacteria. This archive of frozen microbes gives Lenski the ability to replay E. coli’s tape of life from any point he wishes, simply by thawing out the samples. Along the way, he can examine how the bacteria change both genetically and in ways that are visible under a microscope. Lenski says, “The whole experiment was set up to test how reproducible evolution was.”
In 11 of Lenski’s flasks, the E. coli cells grew physically larger, but bacteria in one flask divided itself into separate lineages—one with large cells and the other with small cells. “We call them the smalls and the larges,” says Lenski. “They have coexisted now for 50,000 generations.” No other population in the experiment did the same; a historically contingent event seemed to have taken place. Even 26 years later, none of the other E. coli lineages evolved it. In this case, contingency seems to have won out over convergence.
In 2003, another contingent event took place. The number of E. coli in one of the flasks increased to the point where the normally translucent nutrient solution turned cloudy. At first Lenski thought that the flask had been contaminated, but it turned out that the E. coli, which normally just feed on glucose in the solution, had developed a way to consume a different chemical in the flasks, called citrate. After 15 years, or 31,500 generations, just one of the populations was able to consume the substance.2 Its population size quickly expanded by a factor of five.
This “historical contingency” gave Lenski and his graduate student Zachary Blount a chance to examine the likelihood that it would happen again if they rewound the tape. Blount went to the archive of frozen E. coli, and selected 72 samples collected at different periods in the experiment from the population that later evolved citrate metabolism. He thawed them out, and let them grow. Eventually, four out of the 72 samples acquired the ability. What’s more, the mutations only occurred in populations that had been frozen after 30,500 generations. Genetic analysis showed that several genes had undergone mutations that “potentiated” the evolution of citrate metabolism before that point. In other words, the ability to consume citrate was contingent upon other mutations that had come before it. Those formed a fork in the road, altering the path that generations after would be able to travel.
The early, bad mutations were essential to the fitness of later generations, perhaps because they added to the genetic variation that later random mutations could act upon.
The Long-Term Evolution Experiment, as the E. coli project is known, has surpassed 60,000 generations now, giving Lenski a deep data set from which to draw inferences about the interplay of contingency and convergence in evolution. Subtle changes in the bacteria’s DNA that make them larger and better able to proliferate in the flask have been relatively common across the groups. At the same time, Lenski has witnessed “striking” cases of contingency, in which one population did something completely different than the others. But as in convergence, he adds, these transformations weren’t entirely random.
“Not everything is possible,” no matter the process, Wake explains. “Organisms evolve within the framework of their inherited traits.” Organisms can’t pass on mutations that kill them or prevent them from reproducing. In the case of Hydromantes salamanders, their ancestors had to overcome a serious limitation: To acquire their ballistic tongues they had to lose their lungs. That’s because their tongue partly derives from muscles that their predecessors instead used to pump air into the lungs. Now, that formerly small and weak muscle is much larger and stronger. It wraps like a spring around a tapered bone at the back of the mouth, and when the muscle squeezes, the bone generates the force that fires the tongue along with its bones out of the mouth. So, Hydromantes’ ancestor did not simply acquire a mutation and evolve a fast ballistic tongue. Instead, the adaptation followed a series of mutations that first enabled the creature to overcome its reliance on lungs for oxygen and buoyancy control. Each change was contingent on the one before it.
Chameleons, on the other hand, retain their lungs. Instead of re-tooling their lung anatomy, they have evolved a piece of collagen that allows them to catapult their tongues at prey. On the surface, salamander and chameleon tongues converge, but not upon closer inspection. It takes a chameleon 20 milliseconds to shoot its tongue at its prey, a positively glacial pace when compared to the Hydromantes’ five-millisecond firing time. Why are chameleons stuck hunting with such slow tongues? The answer is that they have encountered a kind of obstacle to convergent evolution. The chameleon’s tongue is fast enough to ensure their survival, but they lack the “framework of inherited traits” to evolve the salamanders’ deadlier ballistic anatomy. The chameleons have reached what biologists call an “adaptive peak.”
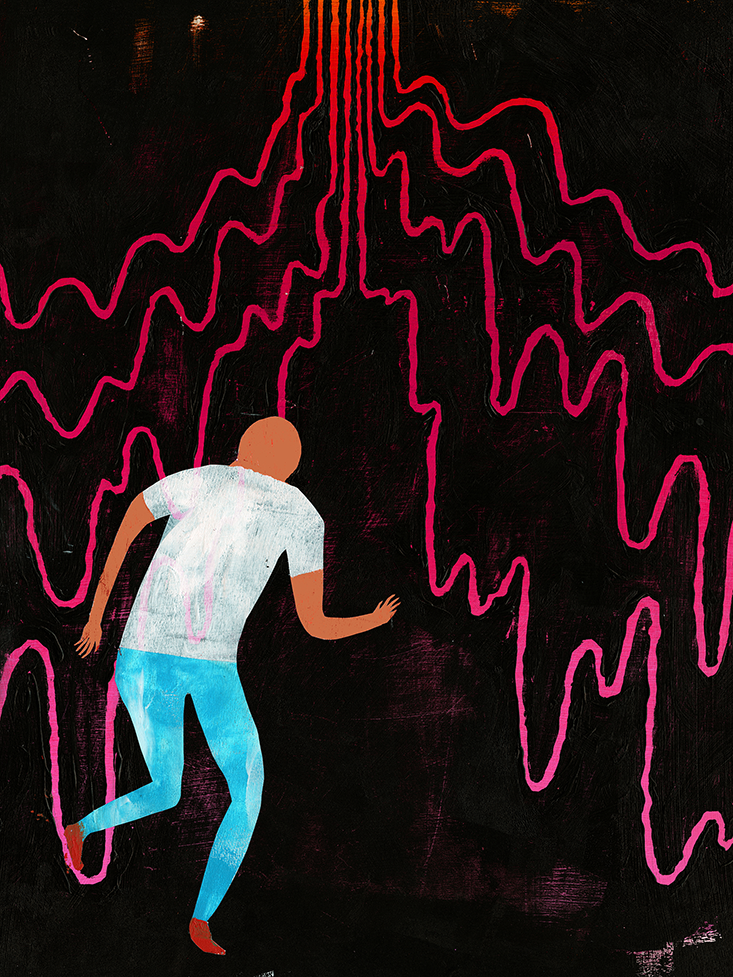
In experiments with viruses that infect bacteria, called bacteriophages, Harvard biologist David Liu discovered adaptive peaks as well. These peaks limit the ability of organisms to converge on a single, optimal design. They help explain why contingencies aren’t often repeated.
Liu wanted to know whether identical groups of bacteriophages could separately evolve a particular enzyme if he put the same pressures on them. He quickened the rate of protein evolution within the viruses, using a system he calls PACE.
During the course of the experiment, the viruses that could not make the enzyme Liu was looking for were removed from the study: Those that achieved the goal, remained. Of those, some had “better” enzymes than others.3 Specifically, the enzyme they made, a polymerase, recognizes a certain DNA sequence and helps turn it into RNA—some polymerases recognized that sequence very precisely and others less so. Like the chameleons’ relatively slow ballistic tongue, these viruses evolved an adaptation that allowed them to survive, but it also prevented them from gaining an even better polymerase. Some viruses were stuck on a lower peak, while a few had climbed to higher ones.
To understand what biologists mean by adaptive peaks, imagine a landscape in which the topography represents the highs and lows of reproductive potential. In the case of Liu’s bacteriophages, different populations searched this landscape through the acquisition of various mutations. Some landed beside small mountains and a few beside Mt. Everest-like peaks. The higher they climbed up their respective mountains, the easier it was to survive. So, they ascended the slope before them. Once at the top of a small peak, viruses could not then move to a taller, more optimal, one. In order to get there, they would need to climb back down, reducing their ability to survive every step of the way. That is a serious challenge because survival of the fittest is a present concern. Which mutation occurs first—which adaptive peak an organism begins to climb—is an historical contingency that convergent evolution can have a very difficult, if not impossible, time overcoming.
What’s true for E. coli is also true for some microbe anywhere in the universe.
The timing of mutations mattered. “Early random events that create differences in the gene pool can have profound effects in determining whether an ultimately beneficial mutation is allowed to effect the survival of an organism,” Liu says. “That randomness erodes the reproducibility of evolution.” In this experiment, contingency had won out over convergence. Past events prevented repeatability.
One way that life may overcome the limitations of adaptive peaks was revealed in studies of digital organisms conducted by Michigan State University computational biologists Chris Adami and Charles Ofria. The duo created a computer program called Avida in which digital organisms evolve under environmental conditions set by the experimenter. Avidians mutate by randomly gaining and losing pieces of code that may allow them to solve math problems, which increases their ability to reproduce.
In one experiment, the Avidians were set to the task of evolving the ability to solve a complex logic problem called “bitwise equals.” Only four out of the 50 digital populations evolved the code necessary to complete the operation.4 All of the successful populations were ones that initially carried a lot of mutations (random pieces of computer code) that made it harder for them to solve math problems and therefore reproduce. It seems counterintuitive, but Ofria found that the early, bad mutations were essential to improving the fitness of later generations, perhaps because they added to the genetic variation that later random mutations could act upon.
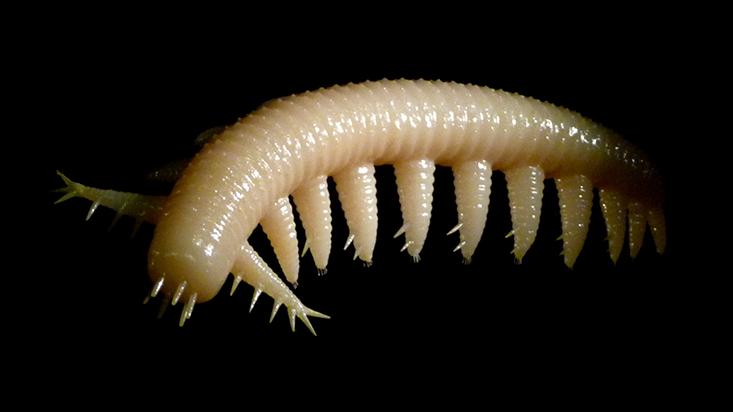
Does the rarity of any particular sequence of events imply that major shifts in evolution are unlikely to be repeated? The experiments suggest that’s true, but Conway Morris firmly answers, no. “You’d be daft to say that there aren’t accidents of one sort or another. The question is one of time scales,” he says. Given enough years and enough mutating genomes, he believes that natural selection will drive life toward the inevitable adaptations that best fit the organisms’ ecological niche, no matter the contingencies that occur along the way. He believes that one day, all of the E. coli in Lenski’s experiment would evolve to consume citrate, and that all of Liu’s viruses would eventually scale their adaptive Mount Everests. Further, those experiments were conducted in very simple and controlled environments that don’t come close to matching the complex ecosystems that life must adapt to outside the lab. It’s hard to say how real-world environmental pressures might have altered the results.
So far, the biggest shortcoming in all of the attempts to answer the “tape of life” question is that biologists can only draw conclusions based on just one biosphere—the Earth’s. An encounter with extra-terrestrial life would undoubtedly tell us more. Even though alien organisms may not have DNA, they’d likely show similar patterns of evolution. They would need some material that would be passed down to their descendants, which would guide the development of organisms and change over time. As Lenski says, “What’s true for E. coli is also true for some microbe anywhere in the universe.”
Therefore, the same interactions between convergence and contingency might play out on other planets. And if extraterrestrial life faces similar evolutionary pressures to life on Earth, future humans may discover aliens that have convergently evolved an intelligence like ours.5 On the other hand, if contingent events build on one another, driving the development of life down unique paths as Gould suggested, extra-terrestrial life may be extraordinarily strange.
Gould believed that humans represented a “wildly improbable evolutionary event,” As evidence, he pointed to the fact that human-like intelligence has only evolved once in 2.5-billion years of life on Earth. He saw the likelihood of another species evolving intelligence similar to our own as vanishingly rare. The idea that we may be the sole sentient species in the universe carries with it some important implications that go beyond biology. “Some find the prospect depressing;” he wrote in Wonderful Life, “I have always regarded it as exhilarating, and a source of both freedom and consequent moral responsibility.”
Zach Zorich is a freelance science journalist and contributing editor at Archaeology magazine.
References
1. Gould, S.J. Wonderful Life: The Burgess Shale and the Nature of History W.W. Norton & Company, New York (1990).
2. Blount, Z., et al. Historical contingency and the evolution of a key innovation in an experimental population of Escherichia coli. Proceedings of the National Academy of Sciences 105, 7899-7906 (2008).
3. Dickinson, B., et al. Experimental interrogation of the path dependence and stochasticity of protein evolution using phage-assisted continuous evolution. Proceedings of the National Academy of Sciences 110, 9007–9012 (2013).
4. Covert III, A., et al. Experiments on the role of deleterious mutations as stepping stones in adaptive evolution. Proceedings of the National Academy of Sciences (2013). Retrieved from: doi:10.1073/pnas.1313424110
5. Conway Morris, S. Life’s Solution: Inevitable Humans in a Lonely Universe Cambridge University Press, Cambridge (2003).