It was nearly midnight aboard the research vessel Atlantis. The ship was about a thousand miles west of Costa Rica, where she’d sailed from, hovering over a hydrothermal vent field in the eastern Pacific. Rutgers microbiologist Costantino Vetriani, seated a few feet away from me in the dark control room, radiated energy despite the hour. He peered intently through his glasses at dozens of monitors, occasionally running a hand over his shaved head. On the live video feed from the remotely operated submersible on the bottom, we watched thick black smoke with a scorching temperature of over 350 degrees Celsius billow from a rocky tower a mile beneath us. It was a stunning sight, an underwater pillar releasing a storm of pent-up energy from the dark bowels of the Earth. Vetriani, a trim Italian clad in a T-shirt reading “RNA: The Other Nucleic Acid,” observed the raw power, his dark eyes shining. “A black smoker is a window into hell,” he said with a grin.
In fact, the black smoker may be a window into the eruption of life on Earth. Vetriani is part of a team of scientists who have come to the vents to study the microbes that carpet every surface in and around them. Earlier, in the ship’s library, chief scientist Stefan Sievert, a microbial ecologist at Woods Hole Oceanographic Institution, had outlined the goals of the month-long expedition. He explained that understanding how the microbes survive in the hellish vents—what nutrients they use and at what rates; how quickly they turn vent fluids into living biomass—could give insight into how biological life evolved. I was tagging along and assisting with record-keeping during the work on the bottom, which ran 24 hours a day, save the occasional interruption when the seas were too rough to deploy the ROV safely.
After midnight, as Vetriani and I took in the black smoker, he explained that the hydrothermal vents were “a relic environment, one we believe resembles what the early conditions on Earth might have been. What we’re doing ultimately is trying to understand how life evolved on the planet.” Being aboard the Atlantis allowed me to learn not only about the primeval microbes that live at vents today, but about how organic life may have first arisen in the ocean’s depths. As we watched the thick, hot fluid venting from the mineral chimney, at the timeless place where rock and water meet at the bottom of the sea, it was as if we were looking directly into life’s birthplace.
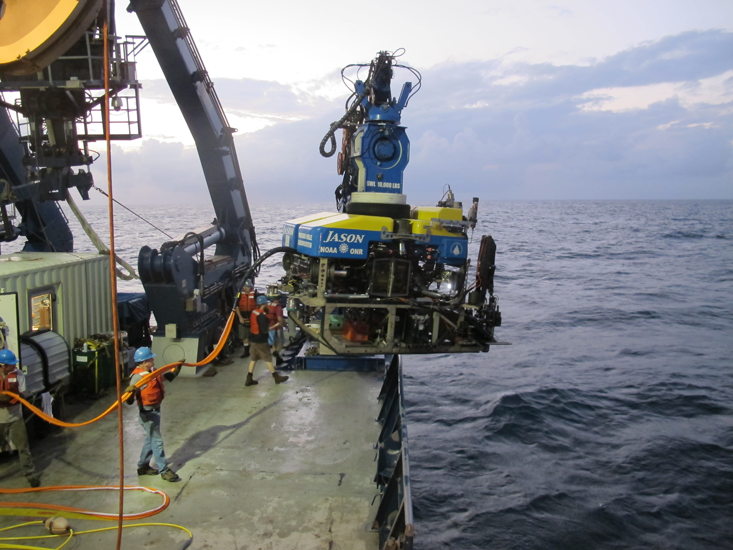
Ever since hydrothermal vents were first spotted by geologists diving in the submersible Alvin in 1977, many scientists have believed these deep-sea crucibles could represent the cradle of life. The matter is still far from settled. But vent fluids and the surrounding water bring together essential elements needed for life, such as carbon, hydrogen, oxygen, nitrogen, and sulfur. This combustible mix, scientists believe, may have spawned the precursors of complex molecules, such as RNA, that stirred to life in the first cells.
Hints of deep-sea vents’ existence came from a 1976 expedition near the Galapagos Islands. An instrument towed near the ocean floor detected a plume of fluid slightly warmer than the cold background seawater. Geologists returned with the submersible the following year and found the smoking seafloor hot springs they’d predicted—along with an utterly unexpected assemblage of living things: clams, giant worms, pink fish, and scuttling white crabs.
The deep sea at the time was thought to be a biological desert, so no one had expected to need any biologists on the vent expedition. But Jack Corliss, a geologist crouched in Alvin during that first dive to the vents, immediately saw them as a possible site for life’s origins. And hydrothermal systems were probably abundant on the early Earth, which was hotter in its youth. Teaming up with John Baross, a microbiologist who studied some of the earliest microbial samples from vents, and graduate student Sarah Hoffmann, Corliss published the hypothesis in 1980. They proposed that simple organic molecules such as amino acids could form in the hot vent fluids, and eventually such molecules could have been enclosed in a membrane to form a living cell. Baross described vents as “the only contemporary geological environment which may be called truly primeval.”
As we watched the thick, hot fluid venting from the mineral chimney, it was as if we were looking directly into
life’s birthplace.
For all of their extremes of temperature, pressure, and other properties, deep-sea vents may have offered a relatively cozy refuge on the violent world of the early Earth. Our young planet was bathed in much stronger ultraviolet radiation from the sun because it hadn’t yet developed a protective ozone layer. That didn’t come along until after the evolutionary invention of photosynthesis pumped a steady supply of oxygen into our atmosphere.
And sunburn wasn’t the only challenge at the surface. Geoscientists think that right around the time that life may have been getting started, a little less than 4 billion years ago, our planet endured a heavy bombardment by space rocks. “That would have made the surface and near-surface lethal,” says Robert Hazen, an astrobiologist at the Carnegie Institution for Science and author of The Story of Earth, which describes the planet’s formation and early life. “But down where these hydrothermal systems occur, it was insulated from that kind of trouble.”
Most important, though, is that vents are bursting with energy. The fluid emanating from them starts out as cold seawater that sinks into cracks and fissures in the seafloor. In the crust, magma heats the water, which picks up dissolved minerals and gases—such as hydrogen and hydrogen sulfide—from the molten rock before shooting back into the ocean. Microbes at vents today use the chemical energy in those fluids to make sugars and other energy-rich molecules that form the base of the food chain.
“Life needs energy,” Sievert said one bright, breezy afternoon on the ship’s bow, in between our morning and evening shifts in the dark control room. “And there’s plenty of it available at hydrothermal systems. So that’s a good place to start. It’s easy to imagine early organisms thriving with that energy source.”
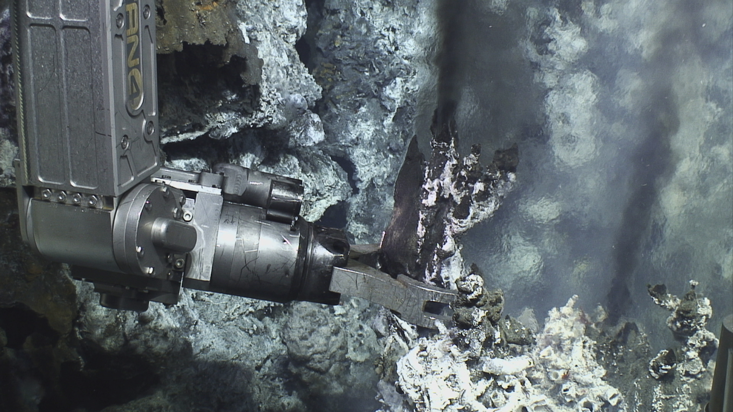
The hypothesis that biological life began in vents got a boost from the discovery of a new kind of hydrothermal vent. In 1991, geochemist Michael Russell, now at NASA’s Jet Propulsion Laboratory, described magnesium rich mineral deposits in Yugoslavia that he suggested had formed at a vent where the fluids were alkaline, in contrast to the acidic stuff typically found at black smokers and other volcanic vents.
No one had ever seen alkaline vents like the ones Russell proposed at the time. Then in 2000, marine geologist Deborah Kelley of the University of Washington and colleagues visited the ridge that runs along the floor of the Atlantic Ocean. They were diving in Alvin every day and towing a camera each night. One night someone noticed a strange white tower on the camera feed. Kelley dove on the site two days later and got the first close look at what she named the Lost City.
Lost City vents form through a chemical reaction between seawater and minerals in iron- and magnesium-rich rocks in the crust. That reaction produces heat energy. As Russell had predicted, it also yields alkaline vent fluids that rise from the seafloor. When those fluids drift up into the ocean, they turn dissolved carbon dioxide into limestone structures that may have housed the beginnings of life.
Origin-of-life scientists perked up as they learned more about the properties of the warm springs of the Lost City. One big attraction is the presence of an ion gradient—a key ingredient in just about every known form of life—between the vent fluids and the seawater. The alkaline fluids are basic, with a pH (a measurement of acidity and alkalinity levels) of around 10 or 11, meaning they have a low concentration of protons. Seawater, with a pH of around 8, is less alkaline—that is, slightly more acidic—so it has more protons than the vent fluids.
That geochemistry caught scientists’ attention because an ion gradient—a difference in the concentration of ions from one place to another—is one of the universal properties of living things and a fundamental part of how cells get their energy. It provides conditions that could be a brewery for early life.
“What just about all of life is doing is producing a proton gradient and then using the flow of those protons across a membrane to generate chemical energy,” says Nick Lane, a biochemist at University College London and author of Life Ascending, about the origin and evolution of life. The general pattern holds true for mitochondria, which are the intracellular generators that power our own cells, as well as for most free-living microbes.
This combustible mix may have spawned the precursors of complex molecules, such as RNA, that stirred to life in the first cells.
The system operates a bit like a hydroelectric dam, Lane explains. A biological pump, in the form of a protein, moves an abundance of protons to one side of a membrane. Then the protons are allowed to flow back across the membrane through another protein that acts as a turbine. The turbine produces ATP, or adenosine triphosphate, the molecule that carries the biochemical energy that powers a cell’s activities.
The specific proteins and membranes vary widely across different forms of life. But the proton gradient is present in just about every life form ever studied. Some researchers see that universality as circumstantial evidence that such a gradient must have been present in the environment where life was born. “It’s very difficult to explain why all of these organisms share this trait unless you can point to some geochemical source of these gradients in the environment where life got started,” says evolutionary biologist Bill Martin of the University of Dusseldorf. “And then, boy do those alkaline hydrothermal vents start to look really good.”
So how might life have bootstrapped its way into existence at a hydrothermal vent like those at the Lost City—or those dotting the Pacific directly beneath the Atlantis? One possible scenario is that reactions between some of the simple chemical compounds at a vent—like carbon dioxide and hydrogen—generated organic molecules, which became increasingly complex.
Essentially, the vent would have acted as a natural hydrothermal reactor. For example, reactions between carbon dioxide and hydrogen, catalyzed by minerals found in the vents, can form a molecule known as pyruvate. Pyruvate is a precursor of many amino acids, which in turn can link together to create proteins. Carbon dioxide and hydrogen can also form formaldehyde, which can react with itself to form ribose, a sugar that’s a component of RNA. Hydrogen cyanide, which has been found at vents, can react with itself to form ringed structures known as bases, another RNA ingredient. Ribose, a base, and a phosphate group (also available at vents) join together to form a molecule called a nucleotide. String several nucleotides together, and you have a strand of RNA. Pores within the vent structures might have played the role of membranes, concentrating the organic molecules such as RNA and amino acids in a small space.
Eventually RNA would begin to self-replicate, a process governed by natural selection. Somewhere along the line, perhaps around 4 billion years ago, the young proto-life inhabiting the vent pores would have acquired DNA, perhaps by making a few chemical tweaks to RNA. (Removing an oxygen atom transforms ribose to deoxyribose, and the addition of a methyl group toggles the single base that differs between DNA and RNA). From there, would-be life would need to assemble a membrane for itself and generate its own ion gradient that could be used to produce energy in the form of ATP. At that point, it could be considered the first cell.
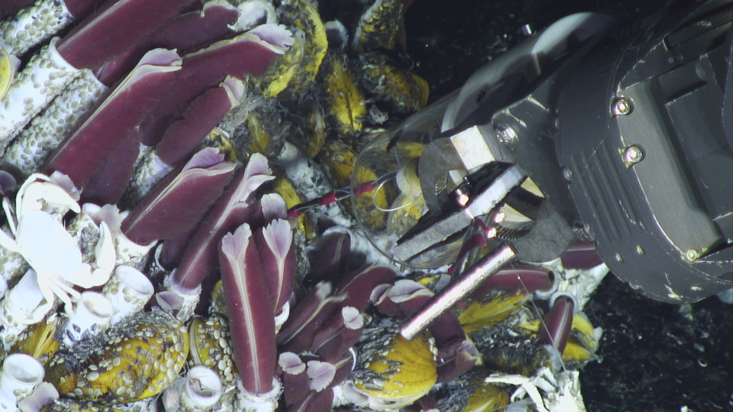
Aboard the Atlantis, a batch of samples from the vents broke the surface, and Vetriani joined the swarm of scientists that descended on it after a crane deposited the haul on deck. He reached into a seawater-filled box and pulled out a black smoker chimney, glittering with pyrite, collected from the bottom, along with a bacterial sampling cylinder made of PVC and mesh. Then he hurried into the ship’s lab, where he and his collaborators began processing the samples.
Many of the microbes that Vetriani and Sievert recovered turned out to be Epsilonproteobacteria, a group of organisms that tend to dominate at vents but not at any other environment on the planet. The pair found that some of these bugs don’t tolerate oxygen and use a metabolic pathway that allows them to “breathe” using sulfur, which is present in abundance at vents. “This sulfur metabolic pathway might have been a core ancestral pathway,” Vetriani said, because it relies exclusively on raw materials thought to have been present on the early Earth and eschews those not thought to have been available.
What concrete evidence did the analysis of these primeval microorganisms provide about life’s origins? Vetriani said he was not comfortable giving an answer. Research into the cradle of life is essentially in its own infancy. “A few decades ago, we didn’t even know life could survive at some of these high temperatures,” Vetriani said. “The origin of life means going back to abiotic processes that might have occurred before the first cell assembled. We’re getting as close as we can by studying these vent organisms to understand what might be those early metabolic pathways. They may be the closest we have to the organisms that might have been living on Earth billions of years ago. The bottom line is the vents are an environment dominated by volcanic activity, which might resemble that of Earth before photosynthesis evolved. We believe that without oxygen, maybe in a volcanic environment, early organisms might have arisen.”
On the ship, as I spent morning after night after morning in the cold, monitor-filled control room, logging what kinds of samples were collected and where, I waited for the sight of the giant smokestacks on the seafloor to grow old, but even after a month, it never did. I’m grimly aware that no matter what happens aboard the Atlantis or in the labs on shore, certainty about the origin of life may always be just out of reach. But now I understand the pull that draws explorers back to this place again and again, hoping each time to get a little closer to our own beginnings.
Lead photograph: A black smoker spews hot, mineral-laden fluid. The containers on the right collect samples of the hydrothermal fluid and any microbes present. Credit: http://www.whoi.edu.
This article was originally published on Nautilus in our “Big Bangs” issue in September, 2014.