Without a doubt, the biggest questions about dreaming are all variants on this question: Why do we dream? We began studying dreaming in the early 1990s and, between the two of us, have published over 200 scientific papers on sleep and dreams. Pulling together a variety of compelling neuroscientific ideas and state-of-the-art findings in the fields of sleep and dream research, we propose a new and innovative model of why we dream. We call this model NEXTUP. It proposes that our dreams allow us to explore the brain’s neural network connections in order to understand possibilities. Think of it as similar to the true goal of education—not to cram facts into our brains, but rather to open us to the unexplored possibilities embodied within those facts, showing us the many ways they can be used and not just one specific way.
Dreaming is a form of sleep-dependent memory processing that extracts new knowledge from existing information through the discovery and strengthening of previously unexplored associations. In doing so, dreams rarely replay active concerns directly or offer concrete solutions to them. Rather, they identify and strengthen associations that in some way embody these concerns and that the brain calculates may be of use in resolving them or similar concerns, either now or in the future.
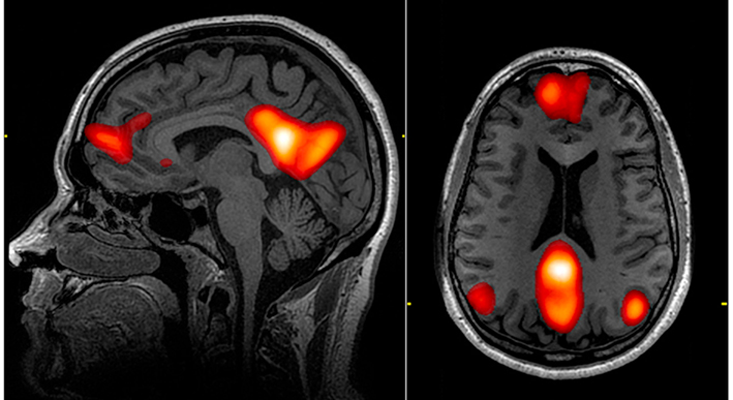
At the same time, dreaming creates narratives that unfold in our minds across time and allows us to experience the thoughts, sensations, and emotions engendered by those narratives. Dreaming, like waking consciousness, allows us to imagine sequences of events, to plan, to plot, to explore. Even when a problem doesn’t inherently require the development of a narrative—for example, figuring out whether adding two odd numbers always produces an even number—we nonetheless create narratives to help us solve them. We “think out loud” about it, “run through it in our mind,” and sometimes go through a series of “steps” as we solve it.
Typically, the brain starts with some new memory, encoded that day—maybe an important event, a discussion overheard at work, or something related to a personal concern—and searches for other, weakly associated memories. These can be from the same day, or they can be older memories from any time in the dreamer’s past. The brain then combines the memories into a dream narrative that explores associations the brain would never normally consider. In doing so, NEXTUP searches for and strengthens the novel, creative, insightful, and useful associations discovered and displayed in our dreams.
Dreaming is a form of memory processing that extracts new knowledge from existing information.
One of us, Bob, measured the brain’s preference for weak associations during REM sleep in a study published back in 1999.1 He used a cognitive test called semantic priming, developed by James Neely at Yale 20 years earlier. It’s a clever test. Participants sit in front of a computer screen as a series of words and non-words, such as “right” or “wronk,” are flashed on it. Their task is simply to respond to each of them by pressing a key labeled “word” or one labeled “non-word.” At the end, Bob calculated how fast and how accurate participants were when responding to the words and non-words. But that’s not the whole story. Before each of these targets was displayed, another word was flashed on the screen for a quarter-second. Depending on the semantic relationship between this “prime” word and the target word (when it was a word), people responded more or less quickly.
Participants identify the word “wrong” faster when it’s preceded by a strongly associated word like “right” than when it’s preceded by a weakly related word like “thief.” And they respond faster in both of these examples than when it’s preceded by a completely unrelated word like “prune.” How much faster someone responds is a measure of their semantic priming. When Bob tested participants during the day, he got exactly the results he expected—strong primes like right produced three times as much priming as weak primes like thief.
But what does it mean? Every time you see a word, your brain activates circuits that allow it to remember the sound and meaning of the word. But it also activates memories of related words. Not only does this activity enable you to understand the word better, but it also prepares the brain for what might be coming next. And the more strongly it activates the memory of a given related word, the faster and more reliably you will be able to identify that word when it does come next. That’s exactly what we’re measuring here. When you respond to wrong a lot faster after a strong prime like “right” (compared to an unrelated word like “prune”), it means your brain strongly activated the target word wrong in response to it. Bob’s results suggested his participants’ brains were activating strongly related words three times more effectively than they were weakly related words.
Bob was able to run this test very quickly; he could get participants through the entire test in just 2 to 3 minutes. This is much faster than the time required for the brain to become fully awake, and for its levels of neuromodulators like serotonin and noradrenaline to shift back to waking levels. By testing participants as soon as they woke up, he was able to ensure that their brains’ neuromodulator levels were still close to where they had been before waking up. He tested them right after waking them from REM sleep in the middle of the night, and the results were better than he could have hoped for. Priming produced by strongly related words dropped by 90 percent, while that produced by weak primes increased more than twofold. When participants were awakened from REM sleep—and presumably while they were in REM sleep just a few minutes earlier—their brains were activating weakly related words eight times more effectively than strongly related words.
When our brains dream, this preference for weak associations helps explain why so many of our dreams lack any transparent connection to the dominant thoughts, feelings, and events of our day. Even when connections are obvious, the usefulness of a dream usually isn’t. But this is exactly what NEXTUP predicts—weakly associated networks are being explored to understand possibilities.
The brain is searching more widely than during wakefulness, going through less obvious associations, and digging for hidden treasures in places it would never consider while awake. In the glare of the day—when our brains are dealing primarily with new incoming sensations and the balance of neurotransmitters in our brain is optimized for processing the here and now—the usefulness, or “rightness,” of these newly found associations might be incomprehensible. But that’s fine. We don’t need to understand why our brain chose these associations. We don’t need to know whether the associations used to construct a given dream were useful. We don’t even need to remember the dream. All the important work was done while we slept. Associations were discovered, explored, and evaluated while we dreamed, and if our brain calculated that some of them were indeed novel, creative, and potentially useful to us, then it strengthened them and filed them away for later use.
Many of our dreams may feel strange and meaningless, but a surprising number of them seem to engender in us a strong sense of their importance. Why does this happen? If the function of dreaming doesn’t require our remembering them, and if we remember so few of them in any case, why should they feel so meaningful when we do? (Indeed, this idea of meaningful dreams is seen across all cultures and across thousands of years.)
We know that the brain is specifically searching for weak associations. This means it is exploring associations that, under normal circumstances, it would reject as somewhere between uninteresting and just plain ridiculous. When we’re dreaming, the brain must shift its bias toward scoring associations as potentially valuable when it normally wouldn’t. It needs to give itself a little push if it’s going to decide that any of the weak associations incorporated into its dream narratives are meaningful and useful.
It’s a little like the ’60s, when people were dropping acid and having profound “acid insights” along the lines of, “When you flush the toilet, everything goes down!” They would tell you this, wide-eyed in awe at their amazing insight, then get a bit sheepish and say, “It meant more than that; it really explained everything.”
The dreaming brain is searching more widely than during wakefulness, digging for hidden treasures.
In fact, the feeling that dreams have meaning is not just a little like the ’60s. It’s likely identical. Pharmacologically, lysergic acid diethylamide (LSD) works by activating the serotonin receptors, including serotonin 1A receptors, which in turn can block the release of serotonin in parts of the brain. All the weirdness of LSD—the hallucinations and acid insights and everything else—may be a direct consequence of this biochemical blockade of serotonin release.
This obviously isn’t the normal state of affairs in the brain. But there is one time every day when serotonin release is completely blocked, and that’s during REM sleep. We dream in both REM and nonREM sleep, but the most bizarre, emotional, and unlikely dreams—and arguably those that seem most meaningful to us—occur in REM sleep. The reduction in serotonin levels during nonREM sleep (relative to wakefulness) and the complete cessation of its release during REM sleep may serve the important role of shifting the brain’s bias toward assigning more value than it otherwise would to those weak associations activated during dream construction. This chemical action may be the grease that enables these potentially useful new associations to slide into our repertoire of valuable insights.
This is just one of the differences in the chemical neuromodulators being released in the brain during sleep. These chemicals control how nerve cells communicate with one another; at the whole brain level, they essentially act to switch the software running the brain. You just learned that serotonin can affect a dreaming person’s sense of how significant a weak association is. When serotonin release is blocked during REM sleep, it leads to an increased sense of wonder and importance for whatever weak association happens to be found. Serotonin release isn’t fully blocked during nonREM sleep, so this bias toward favoring weak associations will be diminished. But that’s just fine, because the brain isn’t looking for weak associations during nonREM sleep.
Bob’s semantic priming experiment showed that our normal preference for strong associations is replaced by one for weak associations during REM sleep. This effect is probably due to a second neuromodulator, noradrenaline, that’s also being shut off during REM. Noradrenaline is the brain’s version of adrenaline; one of its many functions is to focus our attention on what’s right in front of us. You’ve probably noticed that when you’re under pressure and your adrenaline levels skyrocket, you don’t want to think about a bunch of unlikely alternatives to what you are trying to do. You’re so focused, almost nothing can change your course. The disappearance of noradrenaline from the brain during REM sleep makes it easy for your brain to wander among its weak associations.
In recent years, thanks to brain imaging, scientists have discovered the brain’s preference for weak associations during wakefulness, specifically to dreaming’s cousins, daydreaming and mind-wandering. Scientists had long assumed that the activity pattern seen during quiet rest reflected the activity of a brain not doing anything. In retrospect, this was obviously a foolish assumption. Our brains are always thinking about something. The brain areas that turn off when we start to carry out a mental task are the regions that do whatever the brain does when we’re “not doing anything.” Together these regions make up the default mode network (DMN), whose discovery has helped us appreciate just how true it is that the brain never rests.
When we look at the brain regions that make up the DMN, we find a sub-network that monitors the environment for important changes, watching out for any danger. Keeping us safe is probably one function of the DMN. But we also find a sub-network that helps us recall past events and imagine future ones, another that helps us navigate through space, and yet another that helps us interpret the words and actions of others. And these are the mental functions associated with mind-wandering. Much of mind wandering involves hashing over the events of the day or anticipating and planning future events. Indeed, such planning has been proposed as a function of mind-wandering.2 So it’s perhaps not surprising that mind-wandering is associated with increased activity in the DMN.3 This appears to be a second function of the DMN.
When serotonin release is blocked during REM sleep, it leads to an increased sense of wonder and importance.
The DMN is not a static structure, however. It changes based on what you’ve been doing earlier. Bob and his colleague Dara Manoach looked at how activity in the DMN changed after doing one of Bob’s favorite tasks: his finger-tapping task, which involves learning to type the sequence 4–1–3–2–4 as quickly and accurately as possible.4 Young participants get a lot better in just a couple of minutes of practice, but then they plateau. A period of rest in the same day doesn’t make them any faster, but if they get a night of sleep and then try again, they become 15 to 20 percent faster. It’s another example of sleep-dependent memory evolution.
When Bob and Dara had participants learn the task while having their brains scanned, with periods of quiet rest before and after the training, they found that brain regions involved in performing the task were talking to each other more during the quiet rest after training than during the quiet rest before training. The DMN, which is normally measured during such periods of quiet rest, was altered by performing the task. And more important, the more the DMN was altered, the more improvement participants showed the next day. It was as if this new DMN activity was telling the brain what to work on once it fell asleep.
Indeed, much of the DMN is also activated during REM sleep, suggesting that the term daydreaming may be more appropriate than we thought. William Domhoff and his colleague Kieran Fox have gone so far as to suggest that dreaming, or at least REM sleep dreaming, constitutes a brain state of “enhanced mind wandering.”5 More recently, Domhoff has proposed that the neural substrate of dreaming lies within the DMN.6
When you put it all together, you get an exciting extension of our NEXTUP model. Whenever the waking brain doesn’t have to focus on some specific task, it activates the default mode network, identifies ongoing, incomplete mental processes—those needing further attention—and tries to imagine ways to complete them. Sometimes it completes the process shortly after the problem arises, making decisions without our ever realizing it. But at other times it sets the problem aside after tagging it for later sleep-dependent processing, either within or without dreaming. Several dream theories have suggested something like this—that dreaming helps us address areas of concern in our lives. The DMN might provide the mechanism for identifying these concerns, thereby determining how NEXTUP, our Network Exploration to Understand Possibilities, works.
Antonio Zadra is a professor at the Université de Montréal and a researcher at the Center for Advanced Research in Sleep Medicine. He has appeared on PBS’s Nova and BBC’s Horizon. Zadra lives in Quebec.
Robert Stickgold is a professor at Harvard Medical School and director of the Center for Sleep and Cognition. His writing has appeared in Scientific American and Newsweek. Stickgold lives in Cambridge, Massachusetts.
Excerpted from When Brains Dream: Exploring the Science and Mystery of Sleep by Antonio Zadra and Robert Stickgold. Copyright © 2021 by Antonio Zadra and Robert Stickgold. Used with permission of the publisher, W. W. Norton & Company, Inc. All rights reserved.
References
1. Stickgold, R., Scott, L., Rittenhouse, C., & Hobson, J.A. Sleep-induced changes in associative memory. Journal of Cognitive Neuroscience 11, 182–93 (1999).
2. Stawarczyk, D., Majerus, S., Maj, M., Van der Linden, M., & D’Argembeau, A. Mind-wandering: Phenomenology and function as assessed with a novel experience sampling method. Acta Psychologica 136, 370–81 (2011).
3. Schneider, A. & Domhoff, G.W. “DreamBank,” www.dreambank.net; Vandendorpe, C., et al. Base de textes pour l’étude du rêve [Text bank for the study of dreams]. www.reves.ca.
4. Foulkes, D. Dream reports from different stages of sleep. Journal of Abnormal and Social Psychology 65, 14–25 (1962); Rechtschaffen, A., Verdone, P., & Wheaton, J. Reports of mental activity during sleep. Canadian Journal of Psychiatry 8, 409–14 (1963); Fosse, R. Stickgold, R., & Hobson, J.A. Brain-mind states: Reciprocal variation in thoughts and hallucinations. Psychological Science 12, 30–36 (2001).
5. Domhoff, G.W. & Fox, K.C. Dreaming and the default network: A review, synthesis, and counterintuitive research proposal. Consciousness and Cognition 33, 342–353 (2015).
6. Domhoff, G.W. The Emergence of Dreaming: Mind-Wandering, Embodied Simulations, and the Default Network Oxford University Press, New York, NY (2018).
Lead image: agsandrew / Shutterstock