During the winter of 2007, I spent a day half a mile underground. It started with a scary yet exciting ride down the steep incline of a mine shaft, the claustrophobic darkness of the elevator cage aggravated by the din of its steel wheels grinding against the rails. The cage stopped at the 27th level of a disused iron mine in northern Minnesota. The mine, long since abandoned by miners, had been taken over by physicists to search for dark matter, the universe’s presumed missing mass. Inside that mine was the then gold standard of such efforts, the Cryogenic Dark Matter Search (CDMS) experiment. Deep below Earth’s surface, it was shielded from almost every possible source of noise, in the hope that it would “hear” a dark-matter particle hitting one of its detectors.
Even as I admired the physicists’ tenacity, the words of one kept coming to mind. Did such detectors, which were looking for so-called weakly interacting massive particles (WIMPs), even make sense, he asked rhetorically, despite his wholehearted devotion to the project. “Thirty years from now, when one picks up a book on cosmology, the whole WIMP thing could literally be a footnote,” Richard Gaitskell of Brown University in Providence, R.I., told me then. It could turn out that physicists had bet on the wrong horse in their attempts to explain astronomical observations.
Well, 10 of those 30 years have gone by. CDMS became SuperCDMS—larger, quieter, more sensitive. Experimentalists worldwide dug deeper to reduce the noise still further. The Large Underground Xenon experiment, for which Gaitskell is a co-spokesperson, has a mile of rock above it. But apart from the occasional heartbeat-skipping murmurs that turned out to be false alarms, as well as a few highly controversial claims, physicists hear only silence. Searches for other proposed types of dark matter have turned up nothing, too.
Couple this to the other major cosmological conundrum—the nature of the dark energy that is thought to be causing the universe’s expansion to accelerate—and it’s not unreasonable to question the standard model of cosmology, which is built on Einstein’s general relativity and requires both dark matter and dark energy to explain the observed universe. And it’s not unreasonable, either, to think that answers could come from efforts to unify physics. A unified theory will deepen our understanding of gravity, which is the only fundamental force of nature that theorists have not yet reconciled with quantum mechanics. In so doing, it might conceivably account for the galactic motions that we now ascribe to dark matter and dark energy.
The word “quantum” is commonly taken to mean “small,” and indeed conventional wisdom holds that quantum gravity becomes important only over microscopic distances or under extreme conditions, such as in the interiors of black holes. But some physicists suggest that quantum gravity might show up on large scales as well—either because microscopic effects can accumulate over vast distances of billions of light years, or because the theory entails such dramatic changes in how gravity works that it has exotic consequences for galaxies and larger structures.
Take the so-called f(R) theories that date to the 1970s. An attempt to capture the averaged effects of quantum gravity, these theories depart from general relativity in describing how spacetime curves in the presence of matter. The change is subtle and manifests only on the largest cosmological scales (you wouldn’t notice it within our galaxy, for example), but can result in a repulsive force that makes dark energy unnecessary.
If f(R) theories try to do away with dark energy, theories of modified Newtonian dynamics (MOND) do away with dark matter. In this approach, objects respond differently to gravity than Isaac Newton envisaged. This effect becomes important when the acceleration due to gravity falls below a certain threshold, as it does in the outer reaches of galaxies. Effectively, gravity no longer weakens with distance, explaining why stars and gas in the outskirts of galaxies orbit just as fast as they do near the core. Though originally proposed as a modification to Newton’s laws, MOND has since been recast as a refinement of general relativity and perhaps an implication of quantum gravity.
The latest attempt to apply quantum gravity to these problems comes from Erik Verlinde of the University of Amsterdam in the Netherlands, a physicist who has forged a strong reputation in the field of string theory and quantum gravity. With his new theory, Verlinde claims to explain both dark energy and MOND-like behavior in one go. His work builds on a key insight that has emerged over the past decades, which is that spacetime is not a smooth continuum, as Einstein conceived of it, but has a fine-grained structure. No one really knows the nature of this supposed microstructure, and it might well be impossible for us to see directly, but physicists can study its collective properties such as entropy—a measure of its internal disorder. In the ’70s Jacob Bekenstein and Stephen Hawking showed that the entropy of a black hole scales with the exterior surface area of the hole. If this scaling relation is true in general—that is, if the entropy of any given volume of spacetime scales with the surface area of its boundary—then, from it, physicists can derive Einstein’s equations of general relativity.
For his theory of emergent gravity, Verlinde takes the bold leap that the entropy of spacetime has an additional component that scales with volume. His thinking is that our universe, which approximates a spatiotemporal geometry called de Sitter space, is expanding at an accelerated rate, and so has a cosmological horizon—a distance beyond which we cannot see, because galaxies are receding faster than their light can reach us. Such a horizon is very similar to the boundary of a black hole and, by Bekenstein’s and Hawking’s arguments, implies an entropy. This entropy must be counted in addition to the entropy that physicists already ascribe to spacetime, and—crucially, according to Verlinde—it is not localized at the horizon. “The entropy that we normally associate with the horizon should be thought of as entropy that is distributed throughout the de Sitter space,” he says.
To justify his assumptions about entropy, Verlinde appeals to the nature of the spacetime microstructure. The microstructure is highly patterned, so that its parts are mutually correlated. Normally physicists assume that these correlations extend over only very short distances; the properties of two nearby points are very similar, while the properties of two distant points are unrelated. This patterning leads to an area scaling law for entropy. But in de Sitter space, Verlinde argues that the microstructure also has correlations that span large distances. Two distant points may be very similar. Such a patterning implies a volume scaling law.
Sprinkling entropy throughout the volume is tantamount to adding energy to the vacuum of spacetime and raising its temperature. “That energy is dark energy,” says Verlinde. And just like that, the problem of dark energy goes away.
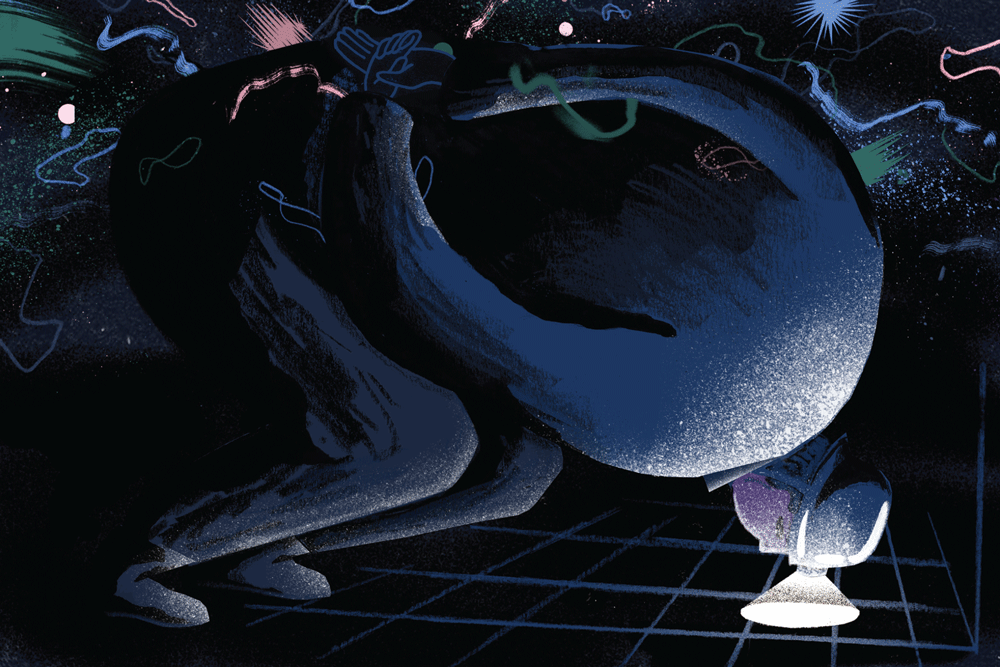
The volume-law assumption also allows Verlinde to explain how galaxies hold themselves together without dark matter. In his theory, when normal matter is added to de Sitter space, it decreases the entropy locally, as if displacing dark energy from the region. According to Verlinde, dark energy responds by pushing back in. “That’s like an elastic force and gives rise to this additional force we are observing,” he says.
Verlinde derives an equation for how this elastic force builds up and how it influences the motion experienced by normal matter in a galaxy, and he finds that the effect becomes apparent—causing deviations from Newton’s laws of motion—when the acceleration falls below a certain threshold, just as MOND theories also say should happen.
One astronomer who has studied MOND in great detail, Stacy McGaugh at Case Western Reserve University in Cleveland, Ohio, is intrigued. “The positive thing to me [about Verlinde’s theory] is that it seems to give a physical reason for MOND-like behavior,” he says. “He’s concerned with some very deep theory of gravity and out of that comes this relation that looks like the data. That’s kind of amazing. There’s no reason to expect that should happen, unless he’s on to something.”
McGaugh has argued for years that certain astronomical observations pose problems for proponents of dark matter, but are naturally explained by MOND. In his most recent work, McGaugh’s team used data from NASA’s Spitzer Space Telescope and the Chandra X-ray observatory to study 240 galaxies of all shapes, sizes, and luminosities. The team mapped both the distribution and the motion of stars and gas (the normal matter) in these galaxies as a function of distance from the galactic center. In a universe with dark matter, you would expect these two sets of measurements to be tightly related in regions dominated by normal matter, close to the galactic center, and loosely related where dark matter calls the shots, farther out.
That’s because, in those outer regions, any relation between the two will be indirect, having to do with how dark matter affects motion and how it affects the distribution of material. Motion is easy: dark matter directly determines the accelerations of the stars and gas. But distribution is complex: many contingent factors come into play. The relative amounts of dark matter and normal matter can vary wildly—by a factor of 100—depending on the type of galaxy, its formation history, and location within the galaxy. So, if you plotted the acceleration of the stars and gas against the distribution of normal matter, you should see a wide scatter in those dark-matter-dominated regions.
Instead, the researchers found that the motion and normal-matter distribution are tightly related everywhere, with very little scatter. Even on the outskirts of galaxies, where dark matter supposedly dominates, there is still a one-to-one correlation between the way normal matter is distributed and the acceleration it experiences, even though this relation doesn’t conform to Newton’s laws. The relation holds across the enormous diversity of galaxies that the team studied. “It’s a dang good correlation,” says McGaugh. It certainly looks as though normal matter can account for the stellar motions; if you were open to modifying Newton’s laws in certain situations, you wouldn’t need to postulate that dark matter provides the additional necessary gravity.
Yet another recent observational study pits Verlinde’s work against dark-matter models. Margot Brouwer of Leiden University in the Netherlands and her colleagues studied the bending of light due to the gravity of galaxies, using a large sample of more than 33,000 foreground galaxies, each of which was bending towards us the light from thousands of background galaxies. Brouwer’s team used estimates of the normal matter in each foreground galaxy, plugged them into Verlinde’s equations, and predicted the gravitational field and the lensing effect of each galaxy. The predictions agreed with observations, within the limits of experimental error.
While both McGaugh’s and Brouwer’s observations can also be explained by models with dark matter, such models need fine-tuning. In both studies, the implied ratio of normal matter to dark matter is not the same for all galaxies and has to be inferred from other observations and simulations. So, purely in terms of explanatory economy, MOND works better. If dark matter is out there, it forces normal matter to behave as if MOND were true, and that has to be explained. Dark matter isn’t just dark, but almost conspiratorial in how it covers its tracks.
All these are challenges for dark-matter models. Astrophysicist Julio Navarro of the University of Victoria in British Columbia, Canada, who is firmly on the side of dark matter, is happy to take them on. “I’m more a member of the establishment, if you want,” says Navarro.
Navarro has an explanation for why dark matter would behave as if MOND were true—why its effects appear below a threshold acceleration and why it gives rise to the one-to-one correlation with normal matter. In the standard model of cosmology, a galaxy is made of stars and gas held together by a massive halo of dark matter. Numerical simulations of galaxy formation show that, if a halo is too big, it cannot host a visible galaxy. So, the acceleration that can be imparted by dark matter in a galaxy is limited. Any time you observe accelerations that are higher—for instance, close to the galactic center—such accelerations can only be due to the additional gravity provided by normal matter. Dark matter governs stellar motion only in the galactic boonies, where the acceleration falls below the threshold value. But even there, normal matter still correlates with the acceleration because the amount of normal matter is fixed by the amount of dark matter. Put simply, MOND is not an alternative law of gravity, but an expression of how dark matter agglomerates.
Navarro acknowledges that such models need additional observations to predict the ratio of normal to dark matter in individual galaxies, but he thinks that’s more to do with not fully understanding the physics of galaxy formation. It does not make him doubt dark matter. Even if you do take this as a strike against dark matter, Verlinde’s emergent-gravity theory has its own failings. For example, Verlinde derived his equations for an idealized case of a spherical mass distribution that is not dynamically interacting with other masses. He has yet to work out in detail the behavior of massive clusters of galaxies, whereas dark-matter models do this relatively effortlessly. Nor is it clear whether Verlinde’s theory can replicate the successes of dark-matter models in explaining features seen in the cosmic microwave background.
Even as observational astronomers try to test Verlinde’s ideas, some theoreticians are also voicing their opinions. Juan Maldacena of the Institute for Advanced Study in Princeton, N.J., objects to the volume law for entropy. “The results of these equations are incompatible with general relativity,” he says. “I have a philosophical problem with this. We should respect the principles of Einstein’s gravity. We should not derive from the equations something that violates the principles.” A handful of theorists that I contacted declined to comment, saying they hadn’t read the paper; in physics, this silent treatment can sometimes be a polite way to reject an idea, although, in fairness, Verlinde’s paper is not an easy read even for physicists.
Even sympathizers think the ideas need fleshing out. “At the current level, [the work] is interesting but not very convincing,” says Sabine Hossenfelder of the Frankfurt Institute of Advanced Studies in Germany. “It needs to be made more rigorous.” That said, she thinks others need to explore the general direction that Verlinde is going in. “There’s a factor of 100 or so more people working on particle dark matter than on modified gravity,” she says. “It is very, very hard for me to tell how much of the success of the [the standard model of cosmology] comes from there just being more people trying to make it work than for modified gravity.”
So, right or wrong, Verlinde’s ideas jolt conventional thinking, says McGaugh. “We need to have a period of chaos, where there are lots of different ideas, and maybe something will turn out to be right,” he says. “But to do that we sort of have to admit that the path we have been on is either wrong or at least inadequate, and that’s a hard thing to do.”
Large sums of money are being spent trying to experimentally verify the standard model of cosmology. I have seen firsthand evidence of these extraordinary efforts in underground mines, in the high-altitude deserts of Chile where telescopes scan the star-littered skies, and in the hostile environments of Antarctica. It’s fair to argue that some fraction of these resources should be devoted to pursuing alterative ideas, whether theoretical or experimental. Our ability to discern the truth about nature should not be held hostage to any one set of ideas.