The brains of human beings are different from those of every other species of animal, because all species’ brains have been tuned to their lifestyles through millions of years of evolution. A spider’s brain is geared to weaving webs and catching flies, a fish’s brain is tuned for a life in the water, and a human brain is geared to human affairs.
But what mental functions separate us most from other animals? What makes us human? Answering this question is a continuous quest of philosophy, comparative psychology, and neuroscience. Many ideas have been put forward, including consciousness, conscience, creativity, sense of self, the ability to remember where and when events in one’s life took place, a sense of fair play and morality, the ability to solve challenging problems, the ability to invent new strategies, the use of tools, and so forth. Most ideas of the distinction between animals and humans have been disputed by naturalists, ethologists, and neuroscientists, who see elephants mourning together, chimps teaching other chimps new skills that spread through the community, lions taking revenge on hyenas, birds having a good idea of what was going on in the minds of other birds, animals inventing ways to get food that is otherwise out of reach (e.g., crows dropping stones into a half-filled cylinder to raise food that is floating on the water surface to an accessible level). These human observers have also witnessed macaque monkeys making economic decisions in a laboratory setting that are concordant with the results obtained from the sophisticated mathematical equations found in textbooks of modern economic theory for rational decision making under various risk and reward situations.
Although arguments about what makes us human may never be fully resolved, language is generally agreed to be one of our most advanced traits as a species. But is language really a human specialty? All animals communicate. Ants leave chemical trails, bees dance to tell one another the distance and direction of nectar sources, and even bacteria signal to one another. Our closest relative, the chimp, shows very sophisticated communication skills. One chimp makes eye contact with another who is approaching and then flings his hand abruptly to the side in a gesture that means “Move away!” This is a gesture that humans also often use. Other gestural commands that seem almost intuitive to us are: “Follow me,” or “Look over there!” Chimps also often accompany gestures with hooting vocalizations. Different hoots can signal alarm, patches of food, community membership, individual identity, sexual interest, and so forth. It is undeniable that chimps have a complex communication system. But language, as we humans know it, is a form of communication that employs syntax, grammatical rules, semantic constructions, and references to theoretical concepts, which can be used to package complex ideas together into a sentence that is sometimes far too long, such as this one.
What mental functions separate us most from other animals? What makes us human?
If sophisticated language is particularly human, how does it feature in the human brain? In 1861, the French physician Paul Broca performed an autopsy on the brain of a 51-year-old patient, nicknamed Tan.1 After an accident at the age of 30, Tan was able to say only one word, and that one word was “Tan.” He could understand language and could answer questions. For example, if you asked him to subtract 9 from 13, he would say “Tan Tan Tan Tan” to indicate 4. Broca found a lesion in Tan’s brain at the back of the lateral side of the frontal lobe of the left cerebral cortex. Broca soon had another patient whose speech had been reduced to just five words. After the autopsy on the brain of the second patient, Broca wrote: “I will not deny my surprise bordering on stupefaction when I found that in my second patient the lesion was rigorously occupying the same site as the first.”2 This condition, where patients can understand spoken language but cannot speak it, is most often associated with lesions in just this part of the brain, now known as Broca’s area. A decade later, another physician, Carl Wernicke, working in Austria, identified another brain area that was complementary in many ways to Broca’s area.3 Now known as Wernicke’s area, when this region is damaged, patients can speak but do not understand speech or written language. When speaking, patients with damage to Wernicke’s area choose the wrong words and do not make sense. For example, if asked about what they had for breakfast, they might respond: “The shoestrings under the old oak tree, singing in the sun, always so noisy, don’t you know?” and think that they were answering appropriately.
Once these regions of the cortex that are involved in producing and understanding language had been identified in the human brain, it became possible to ask whether other primates also have these brain areas. The answer is yes. The structural equivalents of Broca’s and Wernicke’s areas have been identified in monkeys from their positions in the cortex, the specialized types of neurons they contain, their patterns of connectivity with other brain areas, and their functions in making and responding to communications. For example, stimulation of the Broca’s area homologue in macaques causes mouth and face movements similar to those used in speech. Gestural communication in monkeys is associated with activity in Broca’s area, and hearing species-specific calls activates both Broca’s and Wernicke’s areas in monkeys, just as language does in humans. So, these areas exist in nonhuman primates and are connected in ways that could pave the way for language. In humans, however, these areas have expanded, especially on the left side of the brain. The average human brain is 3.6 times larger than the chimp brain, yet the human Broca’s area is almost seven times larger than it is in the chimp.
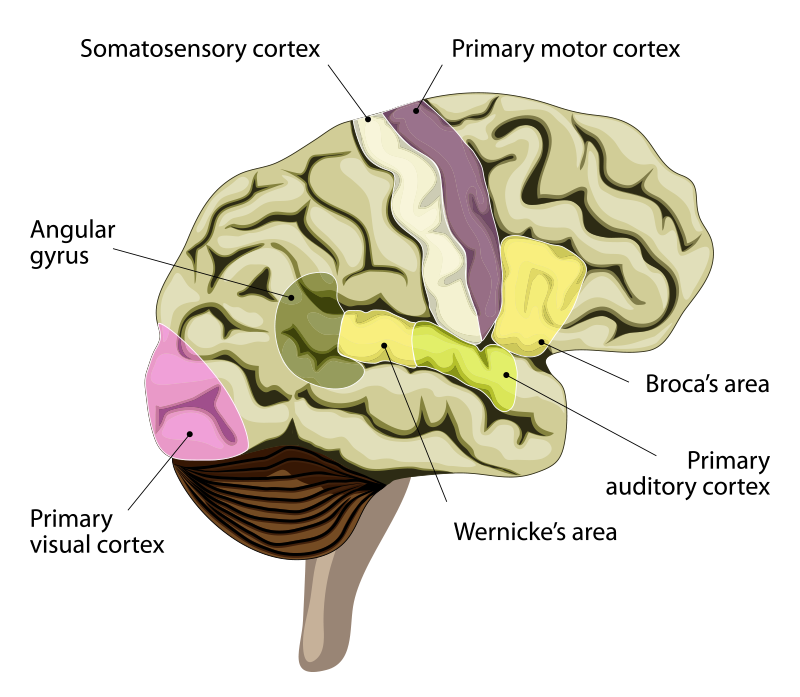
As the language areas of the brain have expanded and specialized in humans through evolution, one might predict that newborn babies already have a disposition for language. Indeed, newborns (three days old and younger) respond to recordings of spoken language more than they do to a language of nonhuman whistles. They also respond better to their native language (the one that they heard in the womb) than to a foreign language, and they respond better when native language is played forward rather than backward—so they already seem to “know” many important things about language. Electroencephalographic and functional magnetic resonance imaging studies show that areas of the left cerebral cortex, where language is encoded and decoded, light up in babies when they hear language. Remarkably, recent research has even shown that a region of the left temporal lobe known as the visual word form area (used for recognizing letters and written words) is already selectively connected to other language centers at birth.4, 5 Though spoken and written language may not be understood or producible for years, regions of the brain are already wired to learn to decode and produce language long before a child is able to speak.
There is a critical period for song learning in zebra finches. Do humans have a similar critical period for learning language? Frequently cited in this regard is the case of an American “feral” child named Genie born in 1957.6 Genie’s child-hating and noise-intolerant father imprisoned her in a room when she was just 20 months old. He strapped her to a toilet during the day, and he strapped her to her bed at night. She was forbidden to communicate with anyone, and she was beaten or denied food when she made any noises. He himself did not speak to her, but instead barked at her, like a dog. She was rescued by the police and hospitalized at the age of 13. Many linguists worked with her until she was 18, which is why this single case is so well documented. Genie became extremely adept at gestural communication, but her language skills improved only a very little.
The implication of Genie’s case is that, like a finch, a human needs to hear and produce language during early life to allow the proper refinement of the neural architecture that can fluently decode and produce language. Without input, the main language centers of the brain atrophy, as scans of Genie’s brain seemed to indicate. Genie’s case is one of an extremely deprived, maltreated, and malnourished child, and so cannot be used by itself to make any solid conclusions. Nevertheless, the idea that there might be a critical period for language acquisition in humans sits well with the rather undisputed fact that almost all adults have more trouble learning second languages than do almost all children and that the youngest children usually have the easiest time becoming fluent and accent-free in a second language.
Variation
Our brains are identifiable as uniquely human through their common anatomical features, yet there is a tremendous amount of variability among human brains.7 Measures of several anatomical features of the cerebral cortex show that human brains are more variable in terms of the shape, size, and thickness of different cortical regions than are those of chimpanzees. Brain size alone in humans can vary up to twofold, and region size commonly varies by this amount. The smallest variability in brain anatomy is found between identical twins. Yet MRI studies of identical twin babies show that differences in patterns of cortical folding can be used to tell them apart with 100 percent accuracy, just as the variations in their fingerprints can be used to tell them apart.8
The same areas of the brain can look similar but function in radically different ways in different people.
Imagine scrunching up a large pizza, which is in fact about the size of an unfolded cortex, to fit inside a human skull. The cerebral cortex scrunches itself up in a coordinated way as it grows. As a result, some of the gyri and sulci (the folds caused by all that scrunching) are identifiable in almost everyone and are consistent enough from individual to individual to have earned names. For example, the central sulcus, which sits near the dividing line between the primary motor and primary somatosensory cortex, separates the frontal lobe from the parietal lobe. But the pizza-scrunching program is not perfectly rigid, so sulci and gyri vary in depth, length, and exact course, and there are many other smaller sulci and gyri along convolutions of the cortex that are so inconsistent that they do not have names.
It is notable that the parts of the brain where the convolutions are most similar among people (e.g., the central sulcus) are closest to the parts of the human cerebral cortex that are most similar in size and shape to those in other primates, and therefore, presumably, the most evolutionarily ancient regions of the cerebral cortex. In contrast, the regions of more variation in the convolutions are found in the higher-level association areas of the cortex (i.e., the regions that have expanded and evolved rapidly in humans). The greater variation in the parts of the cerebral cortex that are newest and are concerned with this higher-level processing may be due to the shorter evolutionary history of these areas compared to the more ancient primary sensory and motor areas.
Experience and Deprivation
The same areas of the brain can look similar but function in radically different ways in different people.9, 10 For example, the visual cortexes of congenitally blind persons become active when they read Braille with their fingers. This does not happen in sighted people. The visual cortexes of blind persons are also active when they hear sounds and language, whereas those of sighted people are hardly activated, if at all, by either auditory or somatosensory inputs.
Studies of the brains of blind children show that their visual cortexes respond well to language as early as the age of 4 years. It seems probable that the visual cortex responds to sound and to touch even at birth, and that over the first few years of life, active visual input eliminates or suppresses these nonvisual inputs in sighted children, whereas they are maintained in children who are blind.
The changes in the visual cortex seen in congenitally blind people stands in contrast to what happens in individuals who become blind in adulthood. The latter fail to show responses to spoken language in their “visual” cortex, even after decades of blindness. Also, when sight is restored to people who were blind as babies, they do not see as well as those whose sight is restored after adult-onset blindness, suggesting that a sensitive period exists during early life when the visual cortex becomes more fixed for other uses in blind people, and it thereby also becomes less able to process and transmit useful visual information. The conclusion one might draw from such findings is that the visual cortex need not be “visual”; it can process other useful information that comes its way. Indeed, this may be true for every area of the cortex. In fact, it may make sense to consider the different regions of the cortex as different processing areas rather than fixed-function or modality-based areas.
The most uniquely human feature of our brain is the expanded size and thickness of the cerebral cortex, which has become, at least anatomically, the predominant neural structure of the human brain. Another feature of the human brain is the remarkable person-to-person variability in cortical lateralization, folding patterns, area sizes, and gray-matter thickness in different regions of the cortex. Some of this variability in the brain—which correlates with differences in personality, cognitive functions, and susceptibility to various neurological and psychiatric syndromes—is accounted for by genetic differences. Other factors, including conditions in the womb, randomizing influences, and early nurturing and childhood experiences, are also involved in shaping the brain.
Finally, beyond childhood, the brain continues to change and update itself through the modification of its synapses. The evolutionary history of the human brain is written in the genome, but the uniqueness of each mind is written in its unique and ever-changing synaptic circuitry. It seems that what makes us all human is also what makes us all different. Everyone’s body and everyone’s brain differs from everyone else’s from the moment of birth, and these differences grow as the final shaping of our bodies and brains is done outside the womb and incorporates our individual experiences in the world. As humans, we can exert control over the environment that provides this experience, so I can leave you with the sobering and perhaps comforting thought that we have some agency in forging the structure, function, and health of the organ that is most critical to our individual identities as human beings.
Adapted from Zero to Birth: How the Human Brain is Built by William A. Harris. Copyright © 2022 by William A. Harris. Reprinted by permission of Princeton University Press.
Lead art: FGC / Shutterstock
References
1. Broca, P. Perte de la Parole: Ramollissement chronique et destruction partielle du lobe antérieur gauche du cerveau. Bulletin de la Société Anthropologique 2, 235–38. (1861). Translated in Berker, E.A., Berker, A.H., & Smith, A. Localization of speech in the third left frontal convolution.” Archives of Neurology 43, 1065–72. (1986).
2. Broca, P. Nouvelle Observation d’Aphémie Produite par une Lésion de la Moitié Postérieure des Deuxième et Troisième Circonvolution Frontales Gauches. Bulletin de la Société Anthropologique 36, 398–407. (1861).
3. C. Wernicke. Der Aphasische Symptomencomplex: Eine Psychologische Studie auf Anatomischer Basis Breslau: Max Cohn & Weigert (1874).
4. Li, J. Osher, D.E., Hansen, H.A. & Saygin, Z.M. Innate connectivity patterns drive the development of the visual word form area. Scientific Reports 10, 18039 (2020).
5. Lai, C.S., Fisher, S.E., Hurst, J.A., Vargha-Khadem, F., & Monaco, A.P. A forkhead-domain gene is mutated in a severe speech and language disorder. Nature 413, 519–23. (2001).
6. Curtiss, S. Genie: A Psycholinguistic Study of a Modern-Day “Wild Child.” Perspectives in Neurolinguistics and Psycholinguistics Academic Press, Cambridge, MA (1977).
7. Toro, R. et al. Genomic architecture of human neuroanatomical diversity.” Molecular Psychiatry 20, 1011–16. (2015).
8. Duan, D., et al. Individual identification and individual variability analysis based on cortical folding features in developing infant singletons and twins. Human Brain Mapping 41, 1985–2003 (2020).
9. Castaldi, E., Lunghi, C., & Morrone, M.C. Neuroplasticity in adult human visual cortex. Neurosci Biobehav Rev 112, 542–52 (2020).
10. Fine, I. & Park, J.M. Blindness and human brain plasticity. Annual Review of Vision Science 4, 337–56. (2018).