Travis Gustavson died in February, 2021 in Mankato, Minnesota at the age of 21. The morning of the day he died, he had a tooth pulled at the dentist’s office. Due to a drug history, the doctors didn’t prescribe him strong painkillers, so he was planning to white knuckle it through the day with ibuprofen, according to his mother. Instead, he called a guy who sold him illegal street heroin and fentanyl. In a text to the dealer, Gustavson sent a photo of the amount he planned to take and asked if he had gotten the dose right. “Smaller bro” and “be careful plz!” the dealer wrote back. Gustavson overdosed.
Gustavson, whose death was reported in the L.A. Times,1 is one of the many casualties of an opioid crisis that has ravaged the United States for over two decades. Opioid overdose deaths have claimed more than 600,000 lives in the U.S. and Canada since 1999, more than were lost in World War I and II combined. In both countries, 2020 was the worst year on record, in terms of total deaths and percentage increase from the previous year, precipitated in part by the anxiety, stress, and isolation of the COVID-19 pandemic.2
Their discovery opened a major new avenue for the treatment of pain.
The urgent need for safer, better painkillers could not be more apparent. In 2016, as the FDA grappled with how to curb the escalating crisis, the agency wrote new rules that limited opioid prescriptions. But given that no powerful clinical alternatives to commonly prescribed opioids exist, these new rules had the unintended effect of driving more people to desperate measures. Some chronic pain patients, after their doses were cut, died by suicide.3 Others sought relief in illict street drugs like heroin and fentanyl.
Though scientists have tried for decades to design drugs that are both more effective and less addictive than opiates, most efforts have run aground.4 At the heart of these failures lies a fundamental misunderstanding of the nature of pain, says Clifford Woolf, a leading expert in pain information processing from Boston Children’s Hospital. It is not a single phenomenon that can be addressed with a universal analgesic,5 but consists of distinct types that demand targeted therapeutic approaches.
Woolf and colleagues at Harvard Medical School are convinced they are finally just a few years away from identifying powerful precision painkillers that could not only safely replace opiates, but effectively target distinct pain types. Their end game is to eliminate chronic pain all together.
Precision Painkillers
In 2006, Alex Binshtok, a young post-doc from Israel by way of Odessa, began a fortuitous collaboration with Woolf, already one of the world’s foremost pain scientists, and neurobiologist Bruce Bean of Harvard Medical School. The three scientists had a bold idea for a way to create targeted painkillers without side effects. Anxious to produce results, Binshtok worked long days in the lab for months, patiently studying the hindpaws of rats, and sleeping among the rodent cages at night.
All neurons express multiple types of sodium, potassium, and calcium ion channels that together regulate their ability to translate stimuli from the external world to the rest of the nervous system. But Woolf, Bean, and Binshtok knew that pain-sensing neurons, called nociceptors, feature unique large-pore ion channels not found on other types of neurons—a discovery made in the late 1990s by David Julius and Ardem Patapoutian, who won the Nobel Prize for related work in 2021. Woolf, Bean, and Bishtok soon identified a way to exploit these unique ion channels to shut down signaling on activated mouse nociceptors, while leaving all other neurons untouched.6
“The big problem with drugs is that they don’t know where to go.”
The implications were vast. Because these compounds worked exclusively on nociceptors that were sensing painful stimuli, they could squelch pain at the source without disabling neurons engaged in other functions. By comparison, most clinically used analgesics tend to hit diverse targets in the central and peripheral nervous systems. Opioids, for example, act not just on nociceptors, but the brain, spinal cord, and the nervous system in the gut, which can lead to sedation, physical dependence, respiratory depression, and death.7
The scientists knew that making the leap from animal models and basic science—the research at the heart of human knowledge—to clinical drug development would be challenging, but they also understood that their discovery opened a major new avenue for the treatment of pain. Within a month, Woolf and Bean submitted their first collaborative paper on ion channel behavior to Nature, where it was published in 2007.6
A decade and a half later, their finding remains a big deal to the field of pain science, says Allan Basbaum, a professor at University of California, San Francisco, who studies the biology of chronic pain. “The big problem with drugs is that they don’t know where to go,” he says. Woolf, Bean, and Binshtok had devised a way to direct drugs to their intended pain targets.
I Need a New Drug
Despite the risks of addiction and overdose, opioids have been used to treat pain for millennia. Egyptian medical papyri from 1550 BC show that healers used poppy plants to cure breast abscesses and to calm crying children, and that tinctures of opium were mixed into eye drops and ointments.8 But over the long term, opioids aren’t an effective treatment for pain, not just because of the potential for deadly side effects, but because the brain demands higher and higher doses to produce the same effect. A recent review published in the Journal of the American Medical Association found that, across a variety of chronic conditions, opioids reduced pain by an average of less than one point on a 10-point scale.9
Less ancient options for pain mitigation—NSAIDs, acetaminophen, ibuprofen, corticosteroids, and anticonvulsant drugs that are prescribed to suppress nerve pain—are clinically effective in only a minority of patients with chronic pain and are also known to have deleterious effects on cells that are unrelated to their pain targets, including cardiovascular tissue.
In 2006, as Binshtok studied his rats, drug development had come to a standstill. Pharmaceutical companies introduced Cox-2 inhibitors in the late 1990s, only to have them black-boxed or banished from the market after reports of heart attacks and strokes.10 When Merck paid out $4.5 billion in legal settlements related to Vioxx, Big Pharma grew leery of taking further chances.
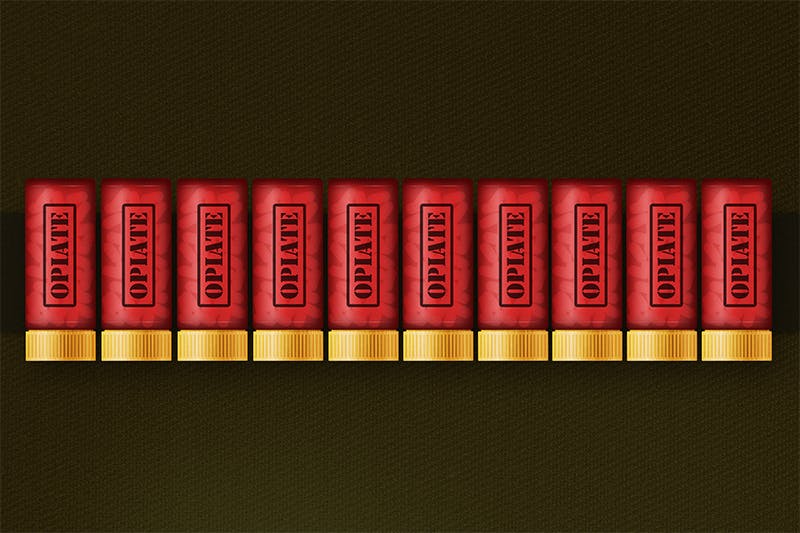
Although few could foresee the oncoming tsunami of opioid-related overdoses and deaths that would unfold over the coming decades, it was already evident that in most developed nations, chronic pain was a serious problem, and that opioid prescriptions meant to treat conditions like bad backs and sore knees were flowing too freely. No one was more aware of this than Clifford Woolf, who had spent much of his career studying the biological mechanisms that underlie chronic pain.
Having revealed, in 1983, the principles behind central sensitization—in which neurons in the central nervous system continue to send pain signals to the brain long after an injury has healed—Woolf spent years searching for therapeutic interventions, compounds that would not carry opioids’ liability for abuse and addiction and whose effects would apply narrowly to a specific target. He found one promising compound in the 1990s, but because it produced psychotic and hallucinogenic effects, it was shelved.11
That failure left Woolf even more determined to find a way to replace highly addictive opioids with safer, better drugs.
The Varieties of Pain
For many decades, the medical profession saw pain exclusively as a symptom of other underlying problems, and treatment choices were determined only by its intensity: mild, moderate, or severe. But pain is various and multifaceted, which is what makes it so difficult to treat. Causes can include cancer, inflammation or tissue injury, injury or lesions of the nervous system, or, as in central sensitization, abnormal amplification of pain signaling in the central nervous system. Or it can manifest as a combination of these subtypes. In some cases, pain is not just a symptom but the disease itself.5
With the diversity of pain in mind, over the past decade Bean and Woolf have devoted much of their energy to learning about different nociceptor cell types. Nociceptors, specialized peripheral sensory neurons that sense noxious stimuli, were discovered in 1906 by Charles Scott Sherrington, an English scientist who later won the Nobel Prize for related work.12 These neurons convert potentially damaging stimuli into electrical signals—action potentials—that are then relayed to higher brain centers. But different types of nociceptors respond to different types of stimuli. Thus, the nociceptors that send messages notifying us of the onset of appendicitis are distinct from those that make our knees and hips feel as creaky as the Tin Man when we get out of bed.13
The urgent need for safer, better painkillers could not be more apparent.
Woolf and Bean have collaborated in their work on nociceptor types with eminent neurobiologist David Ginty, at Harvard Medical School. Ginty’s research has been focused on the neural circuits that are involved in the perception of touch, in all its subtle variations: how the brain perceives the difference between a warm breeze on the cheek and a blow with a blunt object. Of the 14 to 20 neuron subtypes involved in touch, Ginty has found six sensory neurons (thus far) that are specifically tied to pain. These are the nociceptors that Woolf and Bean are studying.
“We study these sensory neurons in great detail,” says Ginty. If they can figure out exactly what kinds of stimuli different types of nociceptors respond to and how they send signals to the spinal cord and the brain, this will help them figure out how to narrowly treat specific kinds of pain. For example, they could selectively inhibit the pain associated with colitis, or with cancer, without blocking pain from the skin, which is protective. Or they could block all nociceptors, and therefore all pain signals, at once, which could be useful during surgery or after major trauma. “That will allow us to consider translational opportunities and think about druggable targets,” says Ginty.
The compounds Woolf and Bean identified in 2007, which they call nocions, are currently being shepherded through clinical trials via a well-funded joint venture called Nocion Therapeutics. But nocions, which can be used to treat pain, cough, and itch, must be administered topically, which limits their therapeutic application. To address the full spectrum of pain, Woolf, Bean, and Ginty are on the hunt for novel analgesic compounds that can work selectively but systemically—drugs that treat specific pain types, but can be taken in pill form.
To really move forward with this work, the three scientists needed a way to eliminate the variables associated with studying rodent models.
In 2006, after the publication of a paper in Nature that explored genetic insensitivity to pain in a Pakistani family with a mutation in the gene SCN9A, which affects the behavior of a specific ion channel,14 pharmaceutical companies invested heavily to develop drugs that targeted that ion channel. The compounds they developed performed very well in rodents. But when those same compounds were tested on humans, the analgesic effect disappeared. “We still don’t really understand why it didn’t work,” says Bean. “It could be that it’s because human nerve cells are larger than those of rats, or that the barrier between blood and nerve cells is different.”
The Harvard scientists knew that they’d have better outcomes if they could conduct their experiments on human nociceptors gleaned from induced pluripotent stem cells (IPSc), which begin as skin or blood cells and can be reprogrammed into any type of cell in the body. This “disease in a dish” model was rapidly becoming the standard in laboratory science, leaving rodent models behind in favor of stem cells in a Petri dish. But despite many attempts, Woolf and Bean had never achieved the enormous supply of high-quality cells required to do large-sale drug screening.
From Dish to Drug—The Long Road Ahead
In February of 2019, at a meeting in Bethesda of the HEAL Initiative (HEAL stands for Helping to End Addiction Long-term) the NIH’s half-billion-dollar plan meant to stem the tide of the opioid crisis, Woolf ran into Don Lo, an old friend from academic scientific circles. Lo, who’d recently been appointed to a key role in drug innovation at the National Center for Advancing Translational Sciences (NCATS), said that instead of heading back to Boston, Woolf would do well to show up at the post-meeting poster session in the NIH cafeteria. There was someone he should meet.
While other attendees chatted over coffee, Illyas Singeç, who directs the Stem Cell Translation Laboratory at NCATS, stood alone in front of a poster covered with unpublished data. After a few minutes of conversation, it was obvious to Woolf that Singeç knew how to produce the high-quality stem cells that he and Bean so desperately needed, at scale. “It was an enormously important moment for pain science. We needed the wheel,” Woolf says simply, “and he had invented it.”
The overriding goal is to eliminate the need for opioids for pain relief.
The first batch of neurons arrived in Boston in June of 2019. Within a few months, in cooperation with a large Harvard Medical School consortium called STOP PAIN,15 Woolf and Bean could do industrial-scale drug screening in an academic setting, making it possible to study hundreds of thousands of compounds. The cells, made at NCATS, were frozen and shipped, and the final stage of the differentiation process was completed in the lab in Boston.
The Harvard consortium, composed of scientists and laboratories from multiple disciplines, gave Woolf and Bean access to a team of medicinal chemists, who could help them select and optimize the most promising drug targets. “We’re the biologists,” Woolf says. “We devise the screens, and when we get a hit—and we’re getting them steadily—the chemists tell us whether it has what it needs to be a drug: that it’s stable and doesn’t break down in a few minutes; that it doesn’t have adverse effects.” STOP PAIN’s computer engineering arm added computational modeling to the arsenal, making it possible for Woolf and Bean to identify the exact molecular and biological mechanisms by which the screened compounds work.
“If I think back to where I was when I started my career,” Woolf says, “the possibilities that are now available and the pace at which the technology advances—what took 20 years, we can now do in two months. It’s an incredibly exciting time to be working in the nervous system. When I began, it was a black box. Now we can begin to identify the way it changes and functions and identify new therapeutic strategies.”
Although Woolf and Bean have a solid head start, the protocol for making these differentiated cells, developed at NCATS, is not exclusive to Harvard’s STOP consortium, or to any organization. Last year, Bean, Singeç, and Woolf published a paper in Science Translational Medicine that details the process of finding nociceptor-selective treatments.16 “Our goal,” Singeç says, “is to disseminate the technology as much as we can, to academic labs and pharmaceutical companies over the next several years.”
Dirty Designer Drugs
Woolf and Bean hypothesize that previous compounds intended to work in ion channels—including ones in which pharmaceutical companies invested millions—failed because they targeted a single channel, allowing another channel on the same cell to step in and do the work of the one that they’d successfully disabled. For that reason, the investigators are designing intentionally “dirty” drugs—ones that will hit multiple ion channel targets—sodium, potassium, and calcium channels that are responsible for different aspects of function on different types of nociceptors.
The big win, says Bean, would be to develop a compound that is useful for the treatment of persistent pain. The statistics back him up: A 2021 paper in Pain noted that in 2019, 20.5 percent of U.S. adults suffered from chronically painful conditions.17
Once these drug candidates have been fully evaluated—the scientists believe that this will take two or three years—they’ll be submitted to the FDA for new drug designation, at which point hungry pharmaceutical companies will hopefully scoop them up and shepherd them through the multi-year phases of clinical trials, which for pain and anesthesia drugs routinely cost more than $100 million.18 (For the drug companies, it should be worth it: In 2021, the market for pain drugs was worth $31 billion, with projections of $39 billion in sales by 2029.)19
The overriding goal, Bean and Woolf agree, is to eliminate the need for opioids for pain relief, by developing compounds that are far more effective, and still safe, with no liability for abuse. Bean says that if they’re successful in the next two to three years, it would represent the ultimate achievement in a lifetime of work. But even if they’re not, they will develop a better understanding of the biology, and a better foundation for the next try. “Each time you try, you set the stage—you help scientists with the next step. We’re convinced that selective inhibiting of these cells can be done. It will work.”
Lead image: Drawlab19 / Shutterstock
References
1. Stobbe, M. U.S. Drug Overdose Deaths Top 100,000 in a year for the first time, officials say. Los Angeles Times (2021).
2. Humphreys, K. Responding to the opioid crisis in North America and beyond: recommendations of the Stanford–Lancet Commission. The Lancet Commissions 399, 555-604 (2022).
3. Szalavitz, M. Cracking Down on Opioids Hurts People With Chronic Pain. Vice (2017).
4. Safavi-Hemami, H. Pain therapeutics from cone snail venoms: From Ziconotide to novel non-opioid pathways. Journal of Proteomics 190, 12-20 (2019).
5. Woolf, C.J. Capturing novel non-opioid pain targets. Biological Psychiatry 87, 74–81 (2020).
6. Binshtok, A., Bean, B.P., & Woold, C.J. Inhibition of nociceptors by TRPV1-mediated entry of impermeant sodium channel blockers. Nature 449, 607–610 (2007).
7. Jayakar, S. et al. Developing nociceptor-selective treatments for acute and chronic pain. Science Translational Medicine 13, eabj9837 (2021).
8. Ross, A.M. Poppy and Opium in Ancient Times: Remedy or Narcotic? Biomedicine International 1, 81-87 (2010).
9. Busse J.W., et al. Opioids for Chronic Noncancer Pain: A Systematic Review and Meta-analysis. Journal of the American Medical Association 320, 2448–2460. (2018).
10. Halpern, G.M. COX-2 inhibitors: a story of greed, deception and death. Inflammopharmacology 13, 419-425 (2005).
11. Woolf, C.J. Evidence for a central component of post-injury pain hypersensitivity. Nature 306, 686-688 (1983).
12. Woolf, C.J. Nociceptors–Noxious Stimulus Detectors. Neuron 55, 353-364 (2007).
13. Dubin, A. & Patapoutian, A. Nociceptors: the sensors of the pain pathway. Journal of Clinical Investigation 120, 3760–3772 (2010).
14. Cox, J.J., et al. An SCN9A channelopathy causes congenital inability to experience pain. Nature 444, 894–898 (2006).
15. Jiang, K. Stopping pain: New consortium aims to transform the treatment of pain. Harvard Medical School (2019).
16. Jayakar, S. et al. Developing nociceptor-selective treatments for acute and chronic pain. Science Translational Medicine 13, eabj9837 (2021).
17. Yong, R. J., Mullins, P. M., & Bhattacharyya, N. Prevalence of chronic pain among adults in the United States. Pain 163, e328–e332 (2022).
18. Ledesma, P. How much does a clinical trial cost? Sofpromed (2020).19. Telugunta, R., Srivastava, A. & Sumant, O. U.S. pain management drugs market. Allied Market Research (2021).