What lies beyond all we can see? The question may seem unanswerable. Nevertheless, some cosmologists have a response: Our universe is a swelling bubble. Outside it, more bubble universes exist, all immersed in an eternally expanding and energized sea—the multiverse.
The idea is polarizing. Some physicists embrace the multiverse to explain why our bubble looks so special (only certain bubbles can host life), while others reject the theory for making no testable predictions (since it predicts all conceivable universes). But some researchers expect that they just haven’t been clever enough to work out the precise consequences of the theory yet.
Now, various teams are developing new ways to infer exactly how the multiverse bubbles and what happens when those bubble universes collide.
“It’s a long shot,” said Jonathan Braden, a cosmologist at the University of Toronto who is involved in the effort, but, he said, it’s a search for evidence “for something you thought you could never test.”
The multiverse hypothesis sprang from efforts to understand our own universe’s birth. In the large-scale structure of the universe, theorists see signs of an explosive growth spurt during the cosmos’s infancy. In the early 1980s, as physicists investigated how space might have started—and stopped—inflating, an unsettling picture emerged. The researchers realized that while space may have stopped inflating here (in our bubble universe) and there (in other bubbles), quantum effects should continue to inflate most of space, an idea known as eternal inflation.
The difference between bubble universes and their surroundings comes down to the energy of space itself. When space is as empty as possible and can’t possibly lose more energy, it exists in what physicists call a “true” vacuum state. Think of a ball lying on the floor—it can’t fall any further. But systems can also have “false” vacuum states. Imagine a ball in a bowl on a table. The ball can roll around a bit while more or less staying put. But a large enough jolt will land it on the floor—in the true vacuum.
In the cosmological context, space can get similarly stuck in a false vacuum state. A speck of false vacuum will occasionally relax into true vacuum (likely through a random quantum event), and this true vacuum will balloon outward as a swelling bubble, feasting on the false vacuum’s excess energy, in a process called false vacuum decay. It’s this process that may have started our cosmos with a bang. “A vacuum bubble could have been the first event in the history of our universe,” said Hiranya Peiris, a cosmologist at University College London.
But physicists struggle mightily to predict how vacuum bubbles behave. A bubble’s future depends on countless minute details that add up. Bubbles also change rapidly— heir walls approach the speed of light as they fly outward—and feature quantum mechanical randomness and waviness. Different assumptions about these processes give conflicting predictions, with no way to tell which ones might resemble reality. It’s as though “you’ve taken a lot of things that are just very hard for physicists to deal with and mushed them all together and said, ‘Go ahead and figure out what’s going on,’” Braden said.
Since they can’t prod actual vacuum bubbles in the multiverse, physicists have sought digital and physical analogs of them.
One group recently coaxed vacuum bubble-like behavior out of a simple simulation. The researchers, including John Preskill, a prominent theoretical physicist at the California Institute of Technology, started with “the [most] baby version of this problem that you can think of,” as co-author Ashley Milsted put it: a line of about 1,000 digital arrows that could point up or down. The place where a string of mainly up arrows met a string of largely down arrows marked a bubble wall, and by flipping arrows, the researchers could make bubble walls move and collide. In certain circumstances, this model perfectly mimics the behavior of more complicated systems in nature. The researchers hoped to use it to simulate false vacuum decay and bubble collisions.
At first the simple setup didn’t act realistically. When bubble walls crashed together, they rebounded perfectly, with none of the expected intricate reverberations or outflows of particles (in the form of flipped arrows rippling down the line). But after adding some mathematical flourishes, the team saw colliding walls that spewed out energetic particles—with more particles appearing as the collisions grew more violent.
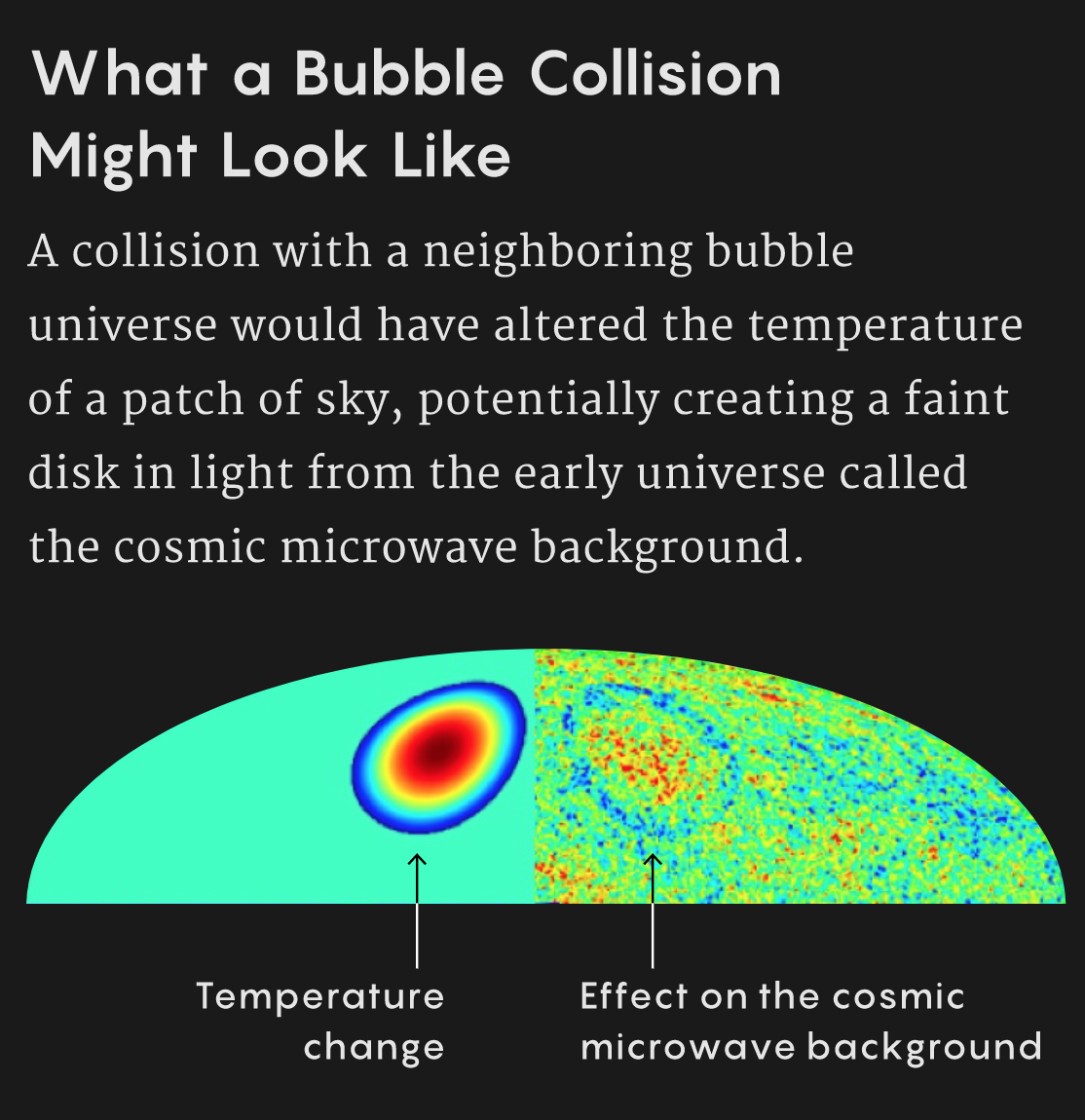
But the results, which appeared in a preprint in December of 2020, foreshadow a dead end in this problem for traditional computation. The researchers found that as the resulting particles mingle, they become “entangled,” entering a shared quantum state. Their state grows exponentially more complicated with each additional particle, choking simulations on even the mightiest supercomputers.
For that reason, the researchers say that further discoveries about bubble behavior might have to wait for mature quantum computers—devices whose computational elements (qubits) can handle quantum entanglement because they experience it firsthand.
Meanwhile, other researchers hope to get nature to do the math for them.
Michael Spannowsky and Steven Abel, physicists at Durham University in the United Kingdom, believe they can sidestep the tricky calculations by using an apparatus that plays by the same quantum rules that the vacuum does. “If you can encode your system on a device that’s realized in nature, you don’t have to calculate it,” Spannowsky said. “It becomes more of an experiment than a theoretical prediction.”
That device is known as a quantum annealer. A limited quantum computer, it specializes in solving optimization problems by letting qubits seek out the lowest-energy configuration available—a process not unlike false vacuum decay.
Using a commercial quantum annealer called D-Wave, Abel and Spannowsky programmed a string of about 200 qubits to emulate a quantum field with a higher- and a lower-energy state, analogous to a false vacuum and a true vacuum. They then let the system loose and watched how the former decayed into the latter—leading to the birth of a vacuum bubble.
The experiment, described in a preprint in June of 2020, merely verified known quantum effects and did not reveal anything new about vacuum decay. But the researchers hope to eventually use D-Wave to tiptoe beyond current theoretical predictions.
A third approach aims to leave the computers behind and blow bubbles directly.
Quantum bubbles that inflate at nearly light speed aren’t easy to come by, but in 2014, physicists in Australia and New Zealand proposed a way to make some in the lab using an exotic state of matter known as a Bose-Einstein condensate (BEC). When cooled to nearly absolute zero, a thin cloud of gas can condense into a BEC, whose uncommon quantum mechanical properties include the ability to interfere with another BEC, much as two lasers can interfere. If two condensates interfere in just the right way, the group predicted, experimentalists should be able to capture direct images of bubbles forming in the condensate—ones that act similarly to the putative bubbles of the multiverse.
“Because it’s an experiment, it contains by definition all the physics that nature wants to put in it including quantum effects and classical effects,” Peiris said.
Peiris leads a team of physicists studying how to steady the condensate blend against collapse from unrelated effects. After years of work, she and her colleagues are finally ready to set up a prototype experiment, and they hope to be blowing condensate bubbles in the next few years.
If all goes well, they’ll answer two questions: the rate at which bubbles form, and how the inflation of one bubble changes the odds that another bubble will inflate nearby. These queries can’t even be formulated with current mathematics, said Braden, who contributed to the theoretical groundwork for the experiment.
That information will help cosmologists like Braden and Peiris to calculate exactly how a whack from a neighboring bubble universe in the distant past might have set our cosmos quivering. One likely scar from such an encounter would be a circular cold spot in the sky, which Peiris and others have searched for and not found. But other details—such as whether the collision also produces gravitational waves—depend on unknown bubble specifics.
If the multiverse is just a mirage, physics may still benefit from the bounty of tools being developed to uncover it. To understand the multiverse is to understand the physics of space, which is everywhere.
False vacuum decay “seems like a ubiquitous feature of physics,” Peiris said, and “I personally don’t believe pencil-and-paper theory calculations are going to get us there.”
Lead image: If our universe is a bubble that inflated inside a larger multiverse, it might bear scars from collisions with nearby bubbles. Credit: Phil Degginger / Science Source