Wearing a headband of replica Tibetan prayer flags, Scott Jurek meets me in a park in Boulder, Colorado to demonstrate proper trail-running technique. This isn’t just jogging down a paved suburban street, but racing along forest and mountain trails that would tax even an unhurried hiker. Last year Jurek ran the entire length of the Appalachian Trail—2,189 miles in 46 days, the equivalent of nearly two marathons every day, over rocky terrain, up and down thousands of feet in elevation.
We walk along a dusty trail segment—while an avid trail runner, I figured I shouldn’t embarrass myself by trying to keep up. What strikes me when I watch him running for demonstration purposes, though, is that Jurek never looks down, no matter how uneven the ground may be. He politely slows pace to stand still and shoot the breeze, waxing rhapsodic about stride rate and length. He calls upon his experience as a health practitioner—he was a physical therapist before he went full-time as an ultrarunner—when discussing matters as complex as neuromechanics and as simple as exhaustion. But for all his knowledge, he isn’t quite sure how he manages to jog these trails without twisting an ankle or falling flat on his face. “Well, you just do it,” he says. “You get in the rhythm, the flow. There’s a lot to keep track of, but actually it’s not all that complicated.”
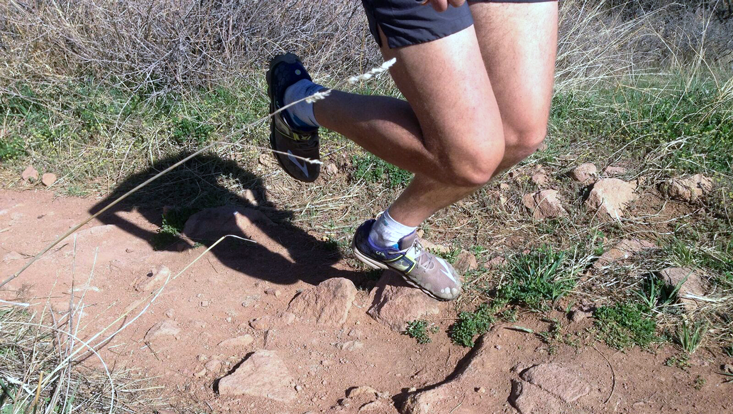
But really, it is complicated. Most biomechanical studies deal with how we negotiate smooth surfaces, perhaps with changes in inclination. Even that is tricky. We are unstable creatures: Both walking and running are a continuous state of imbalance in which one leg supports our body while the other goes through a swinging motion. Somehow our bodies and brains tame the instability and make it productive. Textbooks commonly describe the process using a simple spring-mass model in which our legs are, as physiologist Daniel Ferris from the University of Michigan calls it, “a strikingly consistent set of pogo sticks” (see Sidebar: Pogo Sticks, below). According to this model, running is essentially hopping on two legs. The rate is governed by just a couple of parameters, such as the speed and length of the leg.
Agility relies on cognition that occurs at a high level in the brain, but never breaks into conscious awareness.
The pogo-stick model captures the basic repetitive motion of walking and running, but it poses a puzzle. As the pioneering Russian neurophysiologist Nikolai Bernstein pointed out in the 1930s, the pogo stick moves in a very simple way because of its mechanical design, but the simplicity and fluidity of human movement cannot have such a straightforward explanation. Our muscles can act across our joints in a gargantuan number of ways: Their flexibility creates an enormous problem of control. Those seemingly infinite possibilities for motion, therefore, must be streamlined into a few routinized ones. How do our bodies and brains choose among all the possible courses of action, as if following an intricate trail map that offers multiple means of reaching a destination, and how do they do this in such a consistent way? And how are these choices made when the options expand greatly to include the myriad ways to sidestep a rock on a mountain trail? It seems like too much for the conscious brain to handle. As it turns out, it is.
In 2008 Young-Hui Chang of the Georgia Institute of Technology started attaching springy exoskeletons to the feet of test subjects and watching them hop. Sometimes he wrapped participants in lead strips to simulate their full body weight; sometimes he asked them to hop into spaces marked on the floor, much like the Dance Dance Revolution arcade game. He observed that changes at one joint in our lower limbs are very quickly compensated for by changes in others. The body seems to minimize the control problem by making adjustments only when needed, often with minimal signaling. Ferris, meanwhile, has conducted research that shows something similar using treadmills that resemble jigsaw puzzles: Runners expend more energy on uneven surfaces than on level ones, but the difference is less than theory predicts. Evidently our hips, knees, and ankles become more efficient at moving when terrain becomes complex.
Birds do this too. They are model animals for studying locomotion: They are bipedal, have a human-like gait, and bound over uneven terrain with ease. Monica Daley of the Royal Veterinary College in London keeps a flock of birds and adapted a treadmill for them to run on. She can substitute other materials for the surface of the treadmill, and she finds that the birds’ legs adapt instantly when they touch the material. In one experiment, she used tissue paper that could barely support the weight even of a guineafowl, and yet even as they broke through the paper they recalibrated and ran on anyway, like Jesus walking on water. Other surfaces they tested included sand, foam, and gaping potholes.
The rapidity of their response to new situations intrigues Daley. Guineafowl legs are continuously making fine adjustments. In effect, they have tunable shock absorbers. On uneven surfaces, their legs go into a high-damping mode to reduce the shock of impact; on smooth surfaces, they shift to a low-damping mode to conserve energy. Daley explains: “The benefit of this is greater economy in smooth terrain (when shock absorbers are not engaged), and with the benefit of continued smooth motion in rough terrain (when the shock absorbers are engaged).”
The birds’ response is mechanically mediated—that is, it happens instantly at the point their leg hits the ground, requiring no thought or anticipation at all. Scientists call it a “preflex” to distinguish it from a reflex, which, fast though it may be, still involves external control. The system works, in part, because the natural dynamics of the spring-mass model automatically stabilize the body against disturbances. The central nervous system simply isn’t necessary. Daley points out that even the notorious Mike the Headless Chicken, the remarkable chicken from the 1940s that survived decapitation, could walk.
But such leg-level responses can explain only so much. They adjust for the quality of a surface, but can’t in themselves lead us to evade rocks and roots. For that, we need the brain, which integrates multiple senses: proprioception (our internal bodily awareness), balance, and vision. In other experimental research Daley has conducted with birds ranging from pheasants to ostriches, she has shown that on uneven surfaces birds require neuromechanical feedback, most likely visual in origin, in order to make tradeoffs between stability and leg impact.
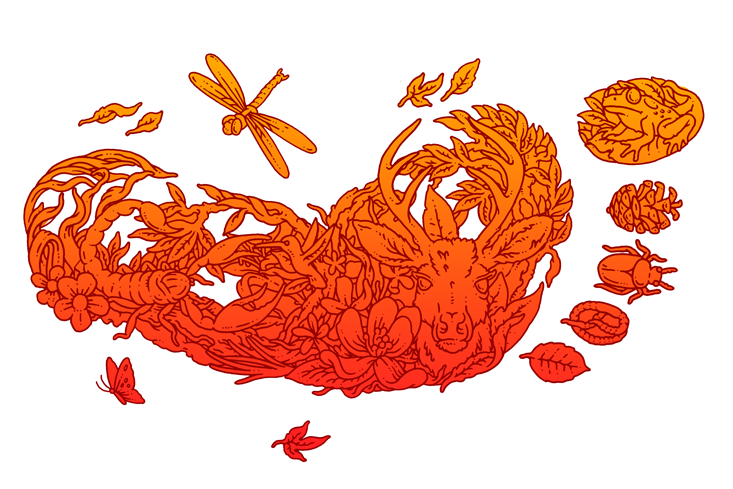
Although our brains help us trail run, it seems clear that our conscious self couldn’t possibly keep up with each tree root and pebble. Interestingly, our visual system—and, with it, other sensory inputs—inform our movement in ways that bypass conscious awareness. “You have to understand that you have two or more visual systems in your brain,” says neuroscientist Christof Koch of the Allen Institute for Brain Science. “One is vision-for-action and the other is vision-for-perceiving. We always get stuck on believing that what we think we don’t consciously perceive isn’t registered by our vision-for-action part of the brain.”
Those two systems are known, respectively, as the dorsal stream (which situates objects in the world and guides motion) and the ventral stream (which identifies what you are looking at and supports conscious awareness). In one demonstration of this divergence, psychologists asked people to grab a moving object and simultaneously utter “tah!” when they noticed its sudden evasion. On average, subjects’ utterances occurred about 420 milliseconds after the fact. Parallel systems process the same visual information for grabbing and for speaking.
In another interesting experiment, psychologists asked research subjects to look at a steep hill and estimate its slope. They gave their answer in one of several ways: Speaking, turning a dial to match the perceived angle, or tilting a board with their hand without looking at that hand. The first two estimates were conscious evaluations: The subjects took in the scene and sought to reproduce what they saw. The third involved a different pathway, from eye to hand, that did not entirely pass through conscious awareness. And the differences were telling. In their conscious estimates, people systematically overestimated the slope, but in their direct eye-to-hand estimates, they got it exactly right. When we decide to plan a motion—a conscious act—our brains often trick us, because geometric precision is less important than, say, the general physical difficulty of the climb. But when we go to execute the motion—unconsciously—we are exquisitely attuned to the environment.
Robots are still flummoxed by environments that humans maneuver without a second thought.
So, when Jurek swears he doesn’t know how he manages to run on a trail, he really doesn’t. His agility relies on cognition that occurs at a high level in his brain, but never breaks into conscious awareness. That high-level functioning is impressive. Jonathan Matthis, a postdoc at the University of Texas, uses motion-capture suits to record the stride of a walking test subject, as well as eye-tracking cameras to precisely measure the subject’s gaze. The data show how they fix their eyes, relative to the phase of their gait. At the moment they actually take the step, they barely use visual information at all. “Walkers make extremely rapid and precise eye movements to gather the visual information they need about the upcoming terrain,” Matthis says, “while simultaneously placing the feet accurately on the basis of information gathered several steps in the past.”
To be sure, our brains do find other ways to integrate the available information. Jurek points out that a blind friend ran with him for part of the Appalachian Trail, and he marvels at his buddy’s foot placement and finely tuned awareness. It’s clear that the nature of our subconscious adaptation and response to noisy terrain is varied—and that all of us do it.
To get a glimpse of it myself, I went to the lab of James Finley at the University of Southern California. A full motion-capture suit, with all its sensors, can take two hours to put on, so I restricted myself to the head cap. Resembling a water polo cap, it monitors infrared light to measure blood oxygenation and volume, a technique called functional near-infrared spectroscopy, or fNIRS.
Finley uses the cap and suit to study how test subjects adapt to new situations. For instance, the good doctor asked me to walk on a treadmill in which the belt was split in two lengthwise to drive each leg independently. He moved the two belts at different speeds to try to throw me off balance, and it kind of worked. I stumbled clumsily. I continued to limp even when, unbeknownst to me, he had switched the belts to a single speed again. The reason, he explained, is that while I was walking with the belts moving at different speeds, my brain adapted its perception of speed to make me feel as though one was moving three times faster than the other. Then, once the belts began moving at the same speed, my brain’s prediction of their speeds was no longer accurate—I began to limp until my brain finally recalibrated. Never mind trail-running: Even walking is a minor miracle.
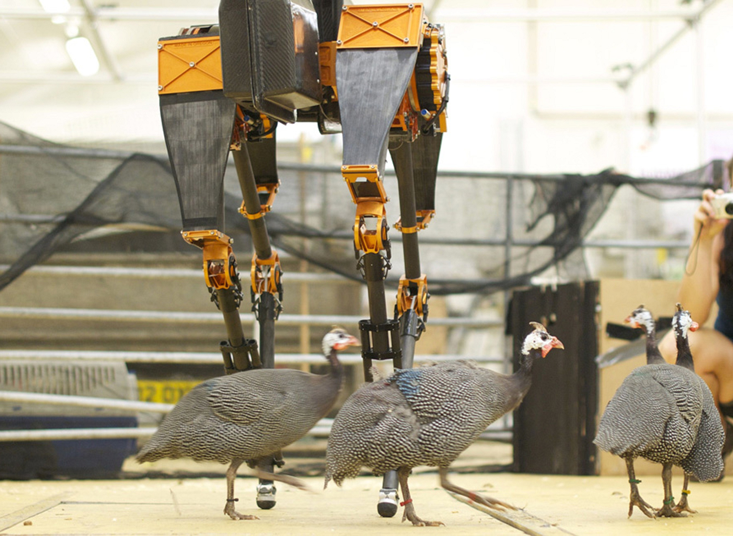
When a robot runs, it doesn’t split the processing work between its conscious and subconscious self. It has neither. That doesn’t mean that robots haven’t taken great strides, in every sense of that term. But they are still flummoxed by environments that humans maneuver without a second thought, failing in many ways because they don’t have the natural elegance to deal with complexity.
“The problem with robots is that they are very good at hopping and all kinds of other locomotion, but they find [running like] the spring-mass difficult, especially on uneven terrain,” laments Hartmut Geyer, a locomotion researcher at Carnegie-Mellon who specializes in robotics. The Atlas robot from Boston Dynamics has recently demonstrated a remarkable ability to stay upright on icy pavement, but the company does not publish its control algorithms. Geyer speculates that they are based on an inverted pendulum, augmented by a flywheel to give the robot some extra maneuverability. The robot faces the same problem of stability as the human body, but, if Geyer is right, solves it in a different way. That said, robots are like humans in that their control systems typically use a combination of simple, fast algorithms, in which sensory input directly affects movement, and slower algorithms that do general planning.
C. David Remy, a roboticist at the University of Michigan, has been particularly interested in the shifting that we undertake in our locomotion. Using robot simulations—and increasingly autonomous bots—he has found that the natural dynamics of human motion are in many ways defined by a frenetic starting and stopping, a switching of velocities that isn’t unlike that of gears in any machine. Our changes in velocity look remarkably jagged—and similar to those of our metallic brethren. Our joints, muscles, and sinews soften the edges of our motion, but if our brains were controlling machine parts rather than organic tissue, our gait would be just as disjointed and jarring as C3PO’s.
Perhaps one day we will design robots that move through a crowded house without getting caught on the rug or running over the cat. And, whether it’s through robotics or human studies, if we could understand how Jurek runs a trail, perhaps we could help people in every part of the movement spectrum, whether it’s elderly people challenged by a walk down the hall, or even pro runners.
After Jurek does a demonstration run for me, deftly making his way down the rocky trail, he stops to wipe off a few dollops of sweat, likely from an earlier morning run, and confesses: “Your body can accomplish a lot, but at the end of the day—like those days where I’d be running for hours on the AT—sometimes you just start stumbling and giving in.”
Sam Schramski is a freelance journalist and researcher currently based in Bloomington, Indiana. He has yet to win a 100 miler but won’t give up until robots start finishing ahead of him.
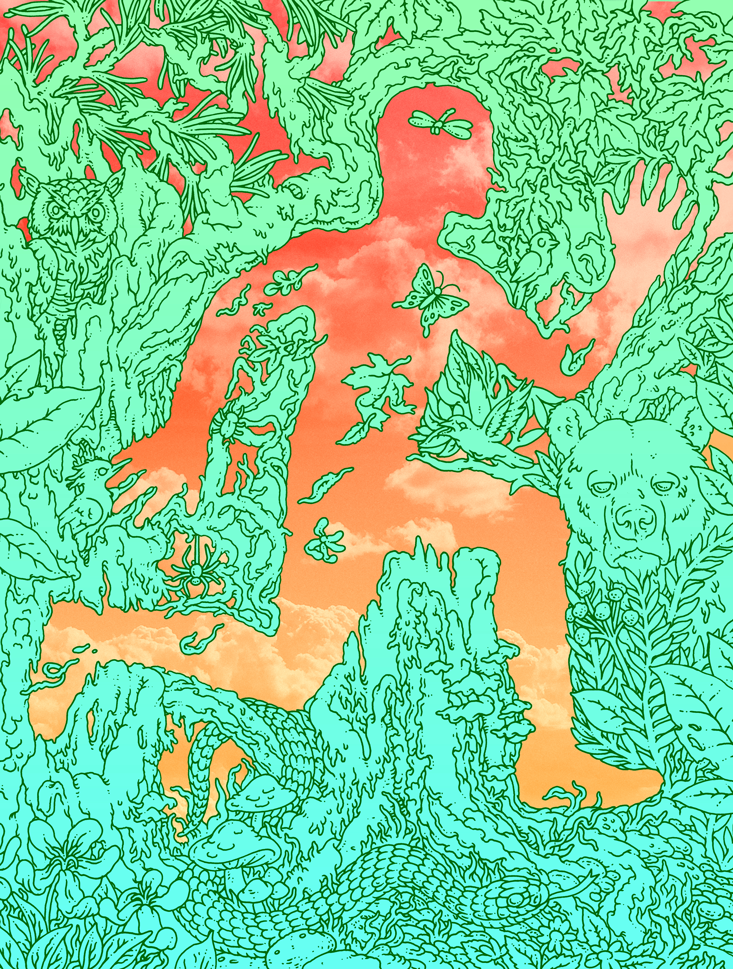
Additional Reading
Birn-Jeffery, A.V., et. al Don’t break a leg: Running birds from quail to ostrich prioritize leg safety and economy on uneven terrain. Journal of Experimental Biology 217, 3786-3796 (2014).
Blickhan, R. The spring-mass model for running and hopping. Journal of Biomechanics 22, 1217-1227 (1989).
Castiello, U., Paulignan, Y., & Jeannerod, M. Temporal dissociation of motor responses and subjective awareness: A study in normal subjects. Brain 114, 2639-2655 (1991).
Chang, Y.H., Roiz, R.A., & Auyang, A.G. Intralimb compensation strategy depends on the nature of joint perturbation in human hopping. Journal of Biomechanics 41, 1832-1839 (2008).
Daley, M.A., Usherwood, J.R., Felix, G., & Biewener, A.A. Running over rough terrain: Guinea fowl maintain dynamic stability despite a large unexpected change in substrate height. Journal of Experimental Biology 209, 171-187 (2006).
Finley, J.M., Long, A., Bastian, A.J., & Torres-Oviedo, G. Spatial and temporal control contribute to step length asymmetry during split-belt adaptation and hemiparetic gait. Neurorehabilitation and Neural Repair 29, 786-795 (2015).
Grabowski, A.M., et al. Running-specific prostheses limit ground-force during sprinting. Biology Letters 6, 201-204 (2010).
Han, J., Waddington, G., Adams, R., Anson, J., & Liu, Y. Assessing proprioception: A critical review of methods. Journal of Sport and Health Science 5, 80-90 (2016).
Koch, C. & Crick, F. The zombie within. Nature 411, 893 (2001).
Matthis, J.S., Barton, S.L., & Fajen, B.R. The biomechanics of walking shape the use of visual information during locomotion over complex terrain. Journal of Vision 15, 10 (2015).
Proffitt, D.R., Bhalla, M., Gossweiler, R., & Midgett, J. Perceiving geographical slant. Psychonomic Bulletin & Review 2, 409-428 (1995).
Sugovic, M., Turk, P., & Witt, J.K. Perceived distance and obesity: It’s what you weigh, not what you think.” Acta Psychologica 165, 1-8 (2016).
Wiesmeier, I.K., Dalin, D., & Maurer, C. Elderly use proprioception rather than visual and vestibular cues for postural motor control. Frontiers in Aging Neuroscience 7 (2015). Retrieved from doi:10.3389/fnagi.2015.00097.
Voloshina, A.S. & Ferris, D.P. Biomechanics and energetics of running on uneven terrain. Journal of Experimental Biology 218, 711-719 (2015).
Xi, W., Yesilevskiy, Y., & Remy, C.D. Selecting gaits for economical locomotion of legged robots. The International Journal of Robotics Research (2015). Retrieved from doi:10.1177/0278364915612572.