Violence has been the sire of all the world’s values,” wrote poet Robinson Jeffers in 1940. “What but the wolf’s tooth whittled so fine the fleet limbs of the antelope? What but fear winged the birds, and hunger jeweled with such eyes the great goshawk’s head?”
We’ve taken these metaphors for evolution to heart, reading them to mean that life is a race to kill or be killed. “Darwinian” stands in for “cutthroat,” “survival of the fittest” signifies survival of the ruthless. We see selective pressures that hone each organism for success and drive genetic innovation as the natural order of things.
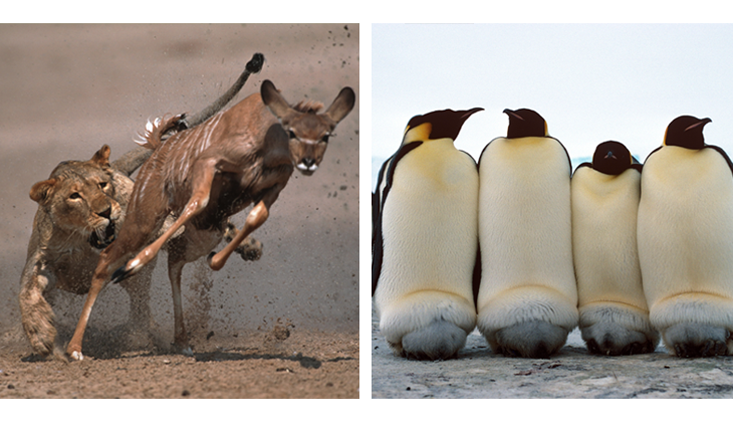
But we know now that that picture is incomplete. Evolutionary progress can be propelled both by the competitive struggle to adapt to an environment, and by the relaxation of selective forces. When natural selection on an organism is relaxed, the creative powers of mutation can be unshackled and evolution accelerated. The relief of an easier life can inspire new biological forms just as powerfully as the threat of death.
One of the best ways to relax selective forces is to work together, something that mathematical biologist Martin Nowak has called the “snuggle for survival.” New research has only deepened and broadened the importance of cooperation and lifting of selective pressures. It’s a big, snuggly world out there.
The fitness of a species can be thought of as a multi-dimensional landscape defined by its compatibility with its environment. The species’ place within that landscape is determined by parameters like its fertility, metabolism, strength, and so on. A “peak” in this landscape represents a place in parameter space where a species’ fitness is high, a “valley” where it could be on the brink of extinction. The slopes of the features are also important. A broad, gentle hill in the fitness landscape would represent an area where the population could mutate and still survive; a narrow ridge would indicate a razor-thin set of possibilities, where even a small change could plunge an individual with a new mutation off a cliff. When selection is relaxed, the fitness landscape itself changes, such that thin precipices broaden out to plateaus. Once a selective constraint is lifted off a trait, the population is able to explore a wider array of possibilities in related traits, and evolution may improvise more freely.
Selection can be relaxed by environmental factors, like a drop in predator numbers. But populations can also relax selection on themselves through their own behavior. A 2017 study conducted by researchers at the University of Sheffield tried to untangle the interplay between behavior and evolution by looking at a decidedly simple behavior in mice: huddling for warmth. The scientists simulated a population of mice, specifying their insulation and metabolic rate, and whether they were loners or huddlers. They tasked an evolutionary algorithm to optimize each population’s metabolic cost while maintaining an ideal temperature range.
The harsh environment didn’t drive the evolution of the behaviors—the behaviors enabled the colonization of harsh environments.
In the case of loners, the solution space allowing mice to efficiently maintain adequate temperatures was tiny. This is doubly problematic for a species. First, if the viable solution space is restricted, it’s much harder for evolution’s random walk to strike upon—“like finding a needle in a haystack.” And secondly, once a solution is found, it’s harder to explore subsequent, potentially beneficial mutations. When a species is inching along a narrow ridge in the fitness landscape, any false step could push it toward extinction. In the researchers’ model, huddling for warmth served to relax selection on the animal’s insulation, allowing genes controlling their metabolism to vary more without compromising their ability to maintain an optimal temperature. This softened the fitness peak, so that successive generations could rapidly explore a broad swath of the fitness landscape and accumulate a greater variety of mutations, providing a richer gene pool that might later be selected for in future times of environmental change. Of course, relaxing selection can also serve to increase the load of potentially deleterious mutations, so there is a tradeoff. But when selection is relaxed and populations are freer to explore the fitness landscape, they may stumble on large adaptive innovations faster.
The authors draw a parallel with the evolution of warm-blooded animals from cold-blooded reptiles. It seems likely that an offshoot of reptiles evolved an insulating factor serving to relax selection—like fur or large body mass—before they began maintaining high body temperatures. Small warm-blooded animals face a great metabolic challenge, as their ratio of surface area to volume is so high that they radiate an enormous amount of heat. Once insulation was in place, the metabolism of proto-mammals was freer to mutate and hit upon stable body temperatures. And when they “discovered” warm-bloodedness, they realized a massive advantage: The first mammals could reliably hunt and forage at night, opening entirely new niches and ultimately resulting in a wildly successful class of animals. The authors argue that by huddling, mice effectively form a “super organism”—sharing heat to behaviorally approximate the benefits inherent to larger organisms without having to evolve a larger body, allowing their metabolism more freedom to change. Of course, computational studies must be taken with a grain of salt—given any model’s requisite assumptions and simplifications—though they allow us to simulate experiments that would take millennia to unfold in nature.
By studying the phylogenetic history of related species, we can begin to correlate the interplay of behaviors with evolutionary dynamics in the real world. This year scientists from Lund University, in Sweden, analyzed the breeding strategies of 4,000 bird species, tracking their movements into new ecosystems using known genetic relationships between the birds. It’s long been known that cooperative-breeding strategies are common in harsh environments. The assumption was that difficult conditions encouraged species to evolve sociable behaviors (at least toward relatives). But what if this presumed causality had it backward? By analyzing the historical migrations of birds, the researchers discovered that species that had already evolved cooperative behaviors in a benign environment were twice as likely to have moved into a harsh one than non-cooperative breeders. The researchers speculate that cooperation buffers against unpredictable breeding seasons, allowing already social populations to be more successful in invading new niches. The harsh environment didn’t drive the evolution of the behaviors—the behaviors enabled the colonization of harsh environments.
We tend to conceive of life as separate from its habitat: The environment is a kind of container, and life is like a liquid that adapts to fill it. Sir Arthur Tansley introduced the concept of the ecosystem in 1935. He believed nature operated like a machine, and so, like an engineer, sought to map the flow of energy and matter through life and its environment. But an ecological niche is not, as I’d gathered from building shoebox dioramas in second grade, the raw physical parameters of an animal’s environment: salinity, alkalinity, humidity, temperature. It’s a web of relations, not just between a species and its habitat, but also with all the other species co-existing in the same space. A niche is no less dynamic than evolution is, contrary to Tansley’s mechanistic vision. “Palaeontologists often say that a burst of diversity in the fossil record simply ‘filled in ecological space,’ as if each new species simply took up residence in a square of a pre-existing chessboard,” writes paleobiologist Douglas Erwin. He suggests that a better analogy is that species build the chessboard themselves. Corals, for example, form their own protective niche by building reefs, which slow currents and reduce erosion on themselves. Reefs also serve to house countless other species, many of which have in turn evolved behaviors to protect corals. If an organism can modify its niche—by altering itself or its relationships with other species—it has the chance to build the world in which its future progeny will evolve, reshaping it to better ensure their survival.
Evolution is not a weapons race, but a peace treaty among interdependent nations.
One striking example of this kind of relationship emerged as a mystery during the dawn of microbiology. In the 19th century, bacteriologists cultured microbes using what was, at the time, cutting-edge technology: a warm vat of meat juice. Physician Robert Koch recognized that such broths likely harbored many different bacterial strains, and he surmised that if the bacteria were given a solid medium on which to grow, different colonies might be separated from each other and studied individually. He split a potato with a sterilized knife and smeared scrapings from an ill patient’s lesions onto it, creating the first solid culture. As different colonies formed, he isolated each onto a separate potato slice, but only a fraction of the divided strains survived alone.
It’s now estimated that 98 percent of bacterial species cannot be singly cultured in a lab, a constraint that is not a purely academic problem: It’s massively hampered the discovery of new biomedical compounds. Our best antibiotics have been stolen from bacteria themselves; after millions of years of co-evolution, many bacteria have evolved highly effective poisons to thwart one another. But if we can’t grow most strains in labs, we can’t isolate the potentially useful compounds they produce. Until 2015, we hadn’t discovered a new class of antibiotic since 1987, and because bacteria evolve so rapidly, many have grown resistant to the antibiotics we’ve employed the past 30 years. There are doubtless many reasons bacteria resist lab life, but chief among them is the fact that, in the wild, bacteria are not self-sufficient: They’ve co-evolved to depend on each other. It may seem precarious, from the vantage point of natural selection, for species to require each other to survive, but the overwhelming ubiquity of interdependence suggest it must have serious advantages. The Black Queen Hypothesis describes one such possibility.
In the Black Queen model, organisms shed genes coding for functions that other species in the environment already provide. It’s a foil to the better-known Red Queen hypothesis, which posits that organisms are subject to a sort of evolutionary arms race, ever adapting new weapons and defenses just to avoid extinction. Though evolution is often characterized as a forward march of complexity, organisms actually shed genes quite often. Biological functions are metabolically costly to maintain, and if they aren’t strictly necessary, they’re best excluded from a genome. (The Black Queen Hypothesis takes its name from the game of Hearts, wherein players try to avoid picking up the queen of spades to avoid a particularly heavy penalty.) A canonical illustration of the Black Queen Hypothesis is found in two free-floating marine cyanobacteria, Synechococcus and Prochlorococcus. They use photosynthesis to feed themselves but are both harmed by a toxic byproduct of the process, hydrogen peroxide. An enzyme that can neutralize hydrogen peroxide, catalase peroxidase, is particularly costly to produce. And, though both need it to survive, only Synechococcus carries the genes for it. Synechococcus mops up all the hydrogen peroxide in the environment, while Prochlorococcus enjoys protection at an energetic discount.
Helper species like Synechococcus can become keystone species in an ecosystem. Because they provide a common good necessary for many species, they may come to be shielded from competition by the species that rely on them, as happens with corals. The success of Prochlorococcus is directly dependent on the relative abundance of Synechococcus. If it begins to outgrow its helper, its numbers will be culled by an increase in hydrogen peroxide. The chessboard has changed: Existence is not a zero-sum game. Shedding the genes for catalase peroxidase confers a substantial energetic benefit to Prochlorococcus, and, as we’ve seen, relaxing selection on a species may allow it to explore new functions in other realms.
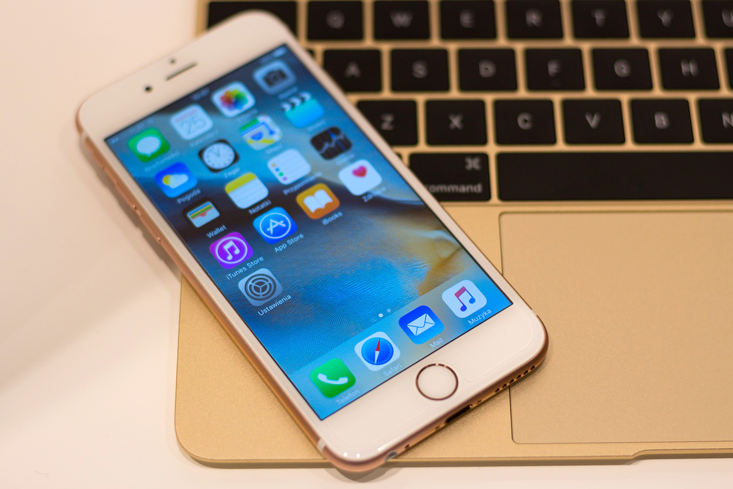
Long periods of harmonious co-existence may be the evolutionary precursor for true symbiotic relationships. Billions of years ago, another ancient cyanobacteria was engulfed and “domesticated” by an ancestor of plants. It shed most of the genes it needed for an independent existence and became what we now know as the chloroplast. In return for a safe environment, these chloroplasts performed photosynthesis for their hosts, fueling a new form of life that eventually spread over much of the Earth. It’s likely this same kind of division of labor was a seed for the development of multicellular organisms. Here, evolution is not a weapons race, but a peace treaty among interdependent nations.
You and I may never have evolved if it weren’t for relaxed selection. Humans have created a unique global niche where we are largely shielded from selective forces: Agriculture staves off starvation, medicine protects us from disease, cultural norms promote group harmony. Our evolution has been profoundly influenced by our selection-buffering behaviors. For instance, the appearance of some modern human features appears to be correlated with a rise in energy consumption, linked to the introduction of meat in our diet. Our ancestor Homo erectus began eating significantly more meat than its predecessors, yet its jaws and teeth were made for crushing tough plant matter and ill-adapted for chewing flesh. This species, it seems, was using tools not only to hunt but also to process meat (and, possibly, using fire to cook it). Energy-rich meat relaxed selection on our metabolism and digestive system—we could devote tenfold less time to chewing vegetation—which paved the way for our modern physiology. Our teeth, jaws, and guts shrank, allowing more energy to be allocated to our swelling brains, which necessitated a protracted, calorie-rich childhood to fully develop. Armed with crude but effective hand axes, Homo erectus shifted its evolutionary destiny. In humans and other animals that learn socially, selection buffering is especially powerful: Adaptive habits, like huddling for warmth and using tools to prepare food, can sweep through a population much faster than genomic changes.
Our genomes continue to be affected by culture to this day. Take the lactase gene, which codes for the enzyme that digests lactose in milk. While it’s present in all human genomes, it has traditionally been turned off after infancy, when children stop nursing. But relatively recently in our natural history, several different groups that farmed cattle evolved the ability to digest lactose throughout their lives, enabling access to a new, valuable form of nutrition. Today it is the descendants of those groups who can drink milk as adults without ill effects.
As humans collected into ever larger groups, the discovery of increasingly complex technology was accelerated. In high-density settlements, artisans and innovators could specialize in their crafts and exchange ideas. Selection for tool development has had an associated pressure on our ability to co-exist peacefully in large numbers, and aggressive, uncooperative individuals may have been selected against. We’ve become, by most accounts, a gentler, more cooperative species over time. Our testosterone levels, for instance, appear to have dropped, judging by the brow size of our fossilized predecessors. Some scientists suggest that the emergence of complex human culture amounts to us having, effectively, domesticated ourselves.
For those most invested in the old-school Darwinian view of the survival of the fittest and violence as virtue, then, the message is clear: Just relax.
Kelly Clancy studies neuroscience as a postdoctoral fellow at the University of Basel, in Switzerland. Previously, she roamed the world as an astronomer and served with the Peace Corps in Turkmenistan. She won the 2014 Regeneron Prize for Creative Innovation for her work designing drug-free brain therapies.
Lead Image: SuperStock / Getty Images