Reprinted with permission from Quanta Magazine‘s Abstractions blog.
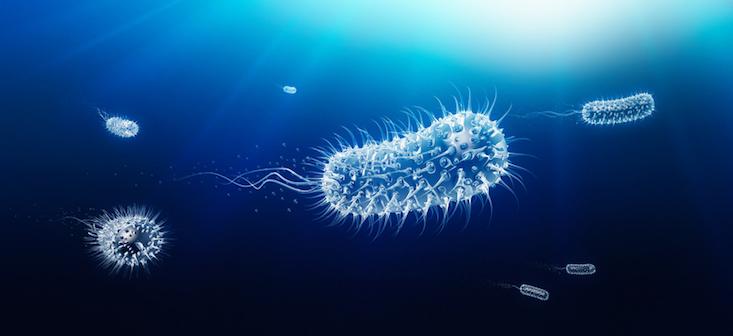
Outside of the imaginations of physics teachers, frictionless devices are hard to come by. But putting a bunch of swimming bacteria into a drop of water achieves just that: a fluid with zero resistance to motion. Incredibly, that resistance (or viscosity, as it’s properly known) can even go negative, creating a self-propelling liquid that might, say, turn a motor in a way that seems to defy the laws of thermodynamics. Recent work explains how bacteria conspire to pull off the improbable.
“For a normal fluid it’s impossible because the whole thing would be unstable,” said Aurore Loisy, a physicist at the University of Bristol in the United Kingdom and a coauthor of one of the new studies, “but for bacteria somehow it works.”
Physicists have long dreamt of getting something for nothing, even if only in outlandish thought experiments. In the 1860s James Maxwell conjured up an all-knowing demon who could shunt fast air molecules to one side of a room and slow molecules to the other, creating a temperature difference that could power an engine. With marginally more practicality, in 1962 Richard Feynman lectured about a microscopic gear that, when jostled by air molecules, would turn in only one direction, driving a motor. But such ideas are dashed by the Second Law of Thermodynamics, which insists that the sorting or the turning must generate heat that dooms both schemes. As the poet Allen Ginsberg put it, you can’t win, and you can’t break even.
Recently, evidence has been mounting that while a free lunch is off the table, a cheap snack might be feasible with a system built around a living fluid. Experimental oddities began to surface in 2015 when a French team confirmed that solutions of E. coli and water could get unnaturally slick. Sandwiching a drop between two small plates, they recorded the force needed to make one plate slide at a certain speed. Liquids usually get harder to stir, or more viscous, when they contain additional suspended particles (think water vs. mud), but the opposite turns out to be true when the particles can swim. When the solution was around half a percent E. coli by volume, keeping the plate moving required no force at all, indicating zero viscosity. Some trials even registered negative viscosity, when the researchers had to apply a bit of force against the plates’ motion to keep them from speeding up. The liquid was doing work, which for any inert fluid would have meant a violation of the Second Law.
The straightforward conclusion was that the organisms were swimming in a way that neutralized the solution’s internal friction to produce something like a superfluid, a liquid with zero resistance. The apparent thermodynamics violation was an illusion because the bacteria were doing the work to offset or overcome the viscosity.
“Each individual bacterium is extremely weak, but there’s strength in numbers,” said Jörn Dunkel, a mathematician at the Massachusetts Institute of Technology who was not involved in the experiment.
If you had enough bacteria in the right setup, you could actually get them to move structures around.
But E. coli don’t typically all swim in the same direction, so subsequent research has tried to figure out what might be coordinating their movements. One answer, according to research published in July in the Proceedings of the National Academy of Sciences, is the interactions between individuals.
“When you have high density, they start to swarm,” said Xiang Cheng, a physicist at the University of Minnesota and coauthor of the paper. But unlike the swarming seen in schools of fish and flocks of birds, the swarming of E. coli is driven purely by their physical characteristics, not an animated response.
The researchers’ setup resembled the French team’s, but an attached microscope allowed them to track the bacteria’s behavior. Sure enough, when the E. coli cocktail reached 10 to 20 percent bacteria by volume, swirls formed. As bacteria plowed through the water, which feels honey-thick at their microscopic scale, they produced shockwaves that buffeted their companions both near and far.
“It’s a bit like if you have a lot of stars in a galaxy and they can affect each other,” Dunkel said. Those forces encouraged local groups of swimming E. coli to align their pill-shaped bodies.
Then the motion of the plates makes that local behavior global. Dragging the top plate sends shearing forces rippling through the fluid, which in effect organize and orient the swarms.
“Without shear, the direction of swarming is random,” Cheng said. “Under shear, you get the tendency to have all the bacteria lining up in certain directions.”
Once the influence of the plates helps the bacteria settle into an average alignment, their swimming pushes on the water and generates local flows that transform the solution’s large-scale properties.
Cheng’s experimental results are largely consistent with a new theoretical model, published just a week earlier in Physical Review Letters. Aiming to develop a mathematical framework to describe the 2015 experiment, the researchers modified equations used for liquid crystals with new terms accounting for the bacteria’s activity.
For a normal fluid it’s impossible because the whole thing would be unstable, but for bacteria somehow it works.
Their theory reproduced the low and negative viscosities seen in experiments and also predicted that the bacteria could collectively orient themselves in multiple stable patterns under the pressure of the plates. “You find that you actually have two possible states, two possible equilibrium solutions,” Loisy said.
Dunkel likened the effect to holding a piece of paper along its top and bottom edges and bringing your hands together: As the paper bends, it folds into either a C or an S shape. It is then unlikely to change from one of those two configurations until released. Cheng’s work also suggests two large-scale orientations, but he expects that both are present simultaneously in different groups of bacteria, and the observed behavior represents an average.
Details about how these effects contribute to the collective superfluidic behavior remain to be worked out, but no one disputes that the transfer of energy from the microscopic to the visible is real, and peculiar. “Usually you cannot do this. You cannot power a motor with a fluid,” Loisy said.
But with bacterial energy, apparently, you can.
“If you had enough bacteria in the right setup, you could actually get them to move structures around,” said Dunkel, which raises the tantalizing possibility of harnessing the plates’ motion to turn a turbine.
In addition to driving a very small motor at bacteria speed, other potential applications include “smart liquids” that could infiltrate underground channels to force out oil or pollutants, according to Harold Auradou, a physicist at the University of Paris-Sud and coauthor of the 2015 paper.
Of course, by all accounts, the laws of thermodynamics remain in full effect.
“You’re not doing anything magic here,” Loisy said.
Two factors let the bacteria solutions succeed where demons and microgears don’t. First, the E. coli act as little engines themselves, metabolizing energy from sugar and oxygen in the water. To keep them moving, researchers take great care to get the balance of nutrients just right. Too little, and they starve. Too much, and they get lazy. “They’re like humans,” Cheng said with a laugh.
But all the energy in the world won’t help if it’s too smoothly distributed, or too disorganized. A system needs asymmetry to coax energy from one location to another. Heat engines require a hot fluid and a cold fluid, for instance, and hydropower turbines need water flowing from a high place to a low place. For bacteria, it comes down to their elongated shape, which responds to the forces in the water.
“Just the fact that they align, that there is a preferred direction, breaks the symmetry,” Loisy said. “If they were spherical it wouldn’t work.”
Charlie Wood is a journalist covering developments in the physical sciences both on and off the planet. His work has appeared in Scientific American, The Christian Science Monitor and LiveScience, among other publications. Previously, he taught physics and English in Mozambique and Japan, and he has a bachelor’s in physics from Brown University.