God might just as well have begun with a toaster oven. A few years ago at a yard sale, Nicholas Hud spotted a good candidate: A vintage General Electric model, chrome-plated with wood-grain panels, nestled in an old yellowed box, practically unused. The perfect appliance for cooking up the chemical precursors of life, he thought. He bought it for $5.
At home in his basement, with the help of his college-age son, he cut a rectangular hole in the oven’s backside, through which an automated sliding table (recycled from an old document scanner) could move a tray of experiments in and out. He then attached a syringe pump to some inkjet printer parts, and rigged the system to periodically drip water onto the tray.
Today the contraption sits atop a workbench in Hud’s laboratory at the Georgia Institute of Technology, where he directs the Center for Chemical Evolution, a multi-university consortium funded by NASA and the National Science Foundation. For the past two decades, he has been hunting for the chemical recipes that could explain how life arose on Earth. When scientists began investigating life’s molecular origin in the 1950s, they assumed that the first biological molecules formed spontaneously from a soup of primordial compounds: a lucky marriage of the right ingredients, under the right conditions, at the right time. Hud and his colleagues are now finding that the spark of life may have struck much more gradually, not by chance but via a long chemical evolution.
The toaster is his latest proving ground. It simulates the cycles of cool and hot, and wet and dry, that Hud suspects jump-started this evolutionary process, millions of years before the first cellular life forms emerged. It mimics dew condensing at night and evaporating with the sunrise; rain puddles filling up and drying out; coastal lagoons flooding and emptying with the tides. Hud calls it the “day-night machine.”
On a spring day last year, he and I are huddled around the homebuilt device, watching it work. Outside of the oven, the syringe delivers a few droplets of water into each of six wells in a ceramic plate on the sliding tray. For the purpose of this demo, the wells are empty; during experiments, they contain a mixture of simple molecules, or monomers, like those believed to have been present on early Earth. The tray disappears into the oven, sealing it shut. As the temperature rises to 185 degrees Fahrenheit (85 degrees Celsius), the water evaporates—the first day. A few minutes pass, and the tray slides out. The wells cool, water drips, and in goes the tray—the second day.
Even if scientists could forge RNA or other fundamental polymers in a test tube, the result wouldn’t reveal their true origin.
Evolution requires two forces: variation and selection. When a brew of monomers dries, the dehydration can induce them to bond together, forming chains called polymers. Wet the mixture again, however, and some of those bonds will break. In other words, the process is reversible. Polymers can form, break down, and form again with new configurations. That’s variation. Eventually, some polymers may stumble upon an advantage. They might fold into shapes that prevent them from breaking apart too quickly, for instance. That’s selection. “These could have been the first survivors of evolution,” Hud says.
Over millions of cycles on primordial Earth, he speculates, some survivors gained other advantages, such as the ability to catalyze reactions. They began to interact and cooperate, forming primitive chemical networks. Sooner or later, some networks started to self-replicate and acquired protective membranes. That’s when life began and biological evolution took the baton. Cell-like sacs begat prokaryotes (bacteria and archaea), begat single-celled eukaryotes (protozoans, slime molds, and algae), begat multicellular organisms (plants and animals), giving rise, at long last, to us.
And if Hud is right, our spinning planet was the giant day-night machine that started it all.
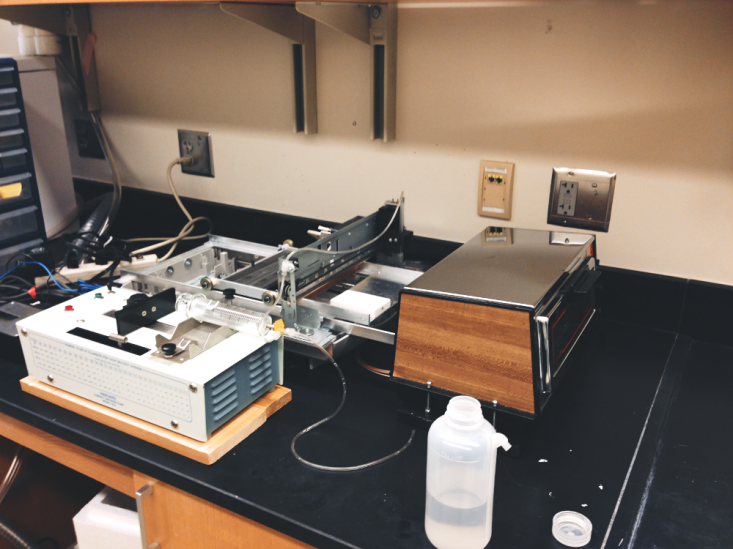
All life depends on two kinds of polymers: proteins, which carry out the basic tasks of metabolism; and nucleic acids (DNA and RNA), which store genetic instructions to be passed on to future generations. In a famous experiment in 1953, chemists Stanley Miller and Harold Urey showed that many of the building blocks for these essential molecules could have assembled on young Earth through chemistry alone, without help from biological processes. By applying an electric spark to a mixture of four primitive compounds—methane, ammonia, hydrogen, and water—they produced amino acids, the basic units of proteins. Using similar methods, researchers later created some of the chemical letters, called nucleobases, that make up the genetic code.
How these simple monomers first stitched themselves into polymers, however, was anyone’s guess. In cells, proteins perform the intricate job of manufacturing and copying DNA, while DNA provides the blueprint for building proteins. In light of this chicken-and-egg paradox, some researchers nominated RNA, which carries information from DNA to protein factories, as the first biological polymer. Perhaps this messenger molecule performed the duties of both proteins and DNA before evolution divvied up these roles. In the 1980s, chemists Thomas Cech and Sidney Altman showed that this may indeed have been the case. Working independently, they discovered that some RNAs can catalyze chemical reactions as proteins do, suggesting that the ancient molecules could have copied themselves.
But scientists have so far failed to recreate RNA under prebiotic conditions. The closest they came was in 2009, when a team led by John Sutherland at the University of Manchester published a recipe in the journal Nature for synthesizing from scratch the basic unit of RNA: a nucleobase attached to a sugar phosphate, which together form a nucleotide. It may be that RNA is too complex to have assembled spontaneously, without help from proteins. If that is true, Hud and others say, it must have developed from a more primitive polymer.
Hud compares RNA to a Stradivarius violin: a perfect instrument. “But you don’t start with making the Stradivarius,” he says. “You start off with somebody taking a stick with a piece of string and stretching it into a bow. That’s how I see RNA—as the descendant of a long line of molecules that evolved to give us this perfect molecule, … the pinnacle of polymers.” Even if scientists could forge RNA or other fundamental polymers in a test tube, the result wouldn’t reveal their true origin. Like Dr. Frankenstein’s monster, the stitched-up creations would say little about the evolutionary process that chiseled their likeness over millions of years.
“For all the time that we’ve had consciousness as humans, we’ve wondered where life comes from. It’d be great if we could put together a story that’s scientifically plausible.”
So Hud’s team has instead focused on searching for RNA’s ancestors—molecules that behaved similarly but assembled more readily from the prebiotic environment. The hunt began a decade ago with a list of 100 monomers that are structurally like the nucleobases of RNA. After testing dozens of candidates under various conditions, the researchers finally made a breakthrough. In a 2013 paper, they reported that two monomers, triaminopyrimidine (TAP) and cyanuric acid (CA), assembled into rings, which stacked on top of one another, forming chains about as long as genes.
In a follow-up study, the team showed that TAP could easily bind with a sugar (a notoriously difficult step) to produce a compound resembling part of a nucleotide. When added to CA, the quasi-nucleotides also grouped into gene-like chains. But these weren’t true polymers. Unlike the actual nucleotides of RNA, which are sewn together like beads on a string, these units were only loosely bound, like magnets.
The result, however, was just the beginning. If structures like TAP-CA chains were the prototypes for RNA—the first crude instruments—how then did they become masterpieces?
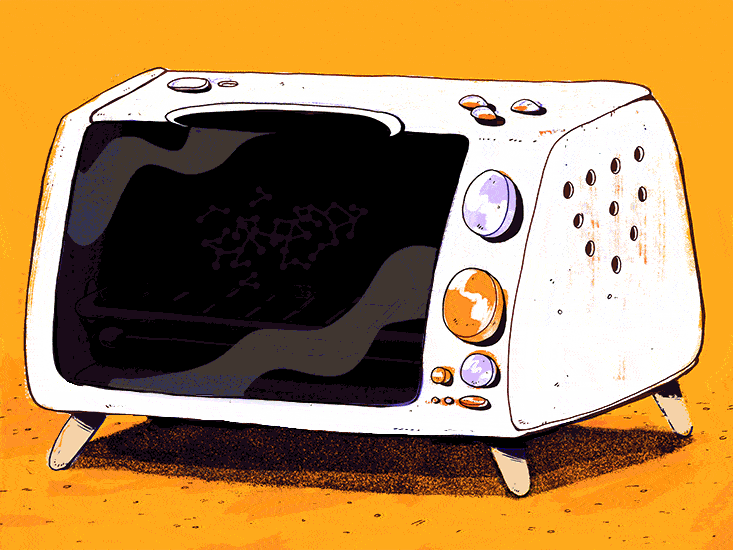
Hud’s office is adorned with scenes inspired by origin stories. On one wall is a replica of Michelangelo’s The Creation of Adam. On another are portraits of the Hindu god Vishnu, perched on the primordial “ocean of milk”, and the Aztec creator Quetzalcoatl, imagined as a feathered snake. “For all the time that we’ve had consciousness as humans, we’ve wondered where life comes from,” Hud muses. “It’d be great if we could put together a story that’s scientifically plausible.”
Hud comes from Los Angeles, where his father was a quality control inspector in the dairy industry. His mother, a nurse and devout Christian, enrolled him in Catholic school for 16 years. But his passion was science. He was a Ph.D. student in biophysics at the University of California, Davis when Cech and Altman won the 1989 Nobel Prize for their discovery of RNA’s protein-like abilities. “That’s all everyone talked about,” Hud recalls. He was hooked.
In 1999, he joined the faculty at Georgia Tech, where he began investigating the possibility that Earth’s cycles—wet and dry, hot and cold, day and night—drove the creation of life’s polymers. In the 1970s, a handful of origin-of-life chemists, including Stanley Miller, had tried to produce proteins and DNA by drying their components on watch glasses in large ovens and then rehydrating them by hand. But they had had little success. Eventually, the approach—never very popular—fell out of use as researchers began looking for life’s origin in locals more exotic than drying puddles, such as hydrothermal vents on the ocean floor.
The engine for life and evolution, Hud says, could be as simple as “a planet spinning in front of a star.”
Hud, however, thought that Miller and his contemporaries had been on the right track. Wet-dry cycles, he realized, could have coaxed polymers into existence by solving what’s known as the “water paradox.” As a solvent, water is vital to life: It facilitates chemical reactions and helps transport nutrients and waste. But in order to make life’s polymers, you have to remove water. Without elaborate cellular machinery to stitch them together, proteins and nucleic acids couldn’t bond in a cell’s watery environment. The first life-like molecules, therefore, must have needed both moisture and desiccation.
Hud decided to start experimenting where his predecessors had left off. But their manual method of wetting and drying monomers proved too tedious. “It quickly became a hassle to do more than two cycles per day,” Hud recalls. “Say we wanted to try 50 cycles—that would have taken almost a month!”
That’s when he decided to build a day-night machine. He tinkered with several designs, including one involving a flatiron and a pizza pan. Another design fitted a robotic arm to a PCR machine, a common lab tool that uses temperature fluctuations to copy DNA. In one of their first successful experiments, he and his team used it to grow chains of primitive compounds called hydroxy acids simply by wetting and drying the monomers, again and again. The cycles acted like a ratchet. When the PCR machine heated a solution of hydroxy acid and water, some of the monomers bonded as they dried. When they cooled and water returned, some of the bonds survived. “The reverse reaction will be slower because we’re at a lower temperature, and so it’s less likely that we’ll break up those bonds,” Hud explains. “We have water coming in, and water going out—back and forth, back and forth. And yet we’re progressing in one direction—we’re building polymers.” His team published their findings in 2014.
In a study published this July, they took the experiment a step further: They added amino acids. With each wet-dry cycle, as the hydroxy acids bonded and grew into polymers, some of the amino acids incorporated themselves into these chains, forming arrangements resembling proteins. Hud suspects that a similar process, perhaps involving the precursors of RNA, likely occurred on early Earth, providing the first step of chemical evolution.
It’s a plausible scenario, says David Deamer, a biophysicist at the University of California in Santa Cruz, who wasn’t involved in the study. The theory is all the more convincing, he adds, because Hud’s team was able to demonstrate polymer formation “without any chemical tricks.”
But to really sell this story, Deamer and others point out, scientists will have to show that wet-dry cycles can not only build polymers but also select for molecules that act as catalysts. Hud’s team created protein-like chains after only 20 cycles. After hundreds—or perhaps thousands—of cycles, will some chains start to contort themselves in new ways? Will they form pockets perfectly shaped to capture another molecule, like a glove fits a hand? Will they then help this molecule react with other molecules, thus orchestrating the chemistry necessary for life?
To answer these questions, Hud will turn to his toaster oven, which offers several advantages over the PCR setup. It’s cheaper and simpler to program, making it easy for his students to run experiments. And it’s versatile. While the PCR machine forces them to test solutions in tiny plastic tubes, the toaster allows for larger volumes of liquids and a variety of surfaces. “We can put any type of object on the tray,” Hud says. “We can feasibly make the whole surface mimic a rock,” a likely birthplace for life. Over the next few months, he plans to use the toaster to ramp up the number of day-night cycles. “The goal is to do over 100 to several hundred cycles,” he says.
If Hud’s team can cycle their way from monomers to catalysts, says Robert Hazen, a mineralogist at the Carnegie Institution, “that would clearly be the mechanism to beat.” The engine for life and evolution could then be, as Hud says, as simple as “a planet spinning in front of a star.”
Johnny Bontemps is a science writer based in Brooklyn, NY.