An elaborate quantum dance powers LED lightbulbs. The more precisely the dance can be choreographed, the closer LEDs will come to fulfilling their promise as the ubiquitous energy-efficient lighting source of the future (LEDs are already efficient, but they could be a lot more so). My latest story, “Mathematicians Tame Rogue Waves, Lighting Up Future of LEDs,” is about a mathematical discovery that allows physicists to plan out that quantum dance step by step, like directors of a Broadway show.
LEDs work when electrons can be coaxed to collide with “holes,” particle-like entities with a positive charge found in semiconducting material. When an electron hits a hole, the LED emits a photon of light.
At least, that’s how it’s supposed to work. In practice, it can be hard to push electrons around with such precision. The semiconducting material used to make most LEDs has a highly messy atomic structure. This means that electrons will sometimes “localize” (or get stuck) before finding a hole. If electrons localize in the wrong place, they emit a phonon of heat instead of a photon of light, and we end up using LED lightbulbs to heat our living rooms.
The mathematical discovery described in my new story should help to solve that problem. Svitlana Mayboroda, a mathematician at the University of Minnesota, and Marcel Filoche, a physicist at the École Polytechnique outside Paris, together with several collaborators, have developed a mathematical formula called the “landscape function” that predicts exactly where electrons will localize. The landscape function allows you to control the localization, Mayboroda said, rather than leaving it to be “given by the gods.”
It can take decades for mathematical discoveries to find a practical application — if they ever do at all. But the landscape function, introduced in 2012, has already been adopted by physicists and engineers. At the University of California, Santa Barbara, the physicists Claude Weisbuch and James Speck have a grant from the Department of Energy to use the landscape function to design a better LED in green — the hardest LED color to engineer efficiently.
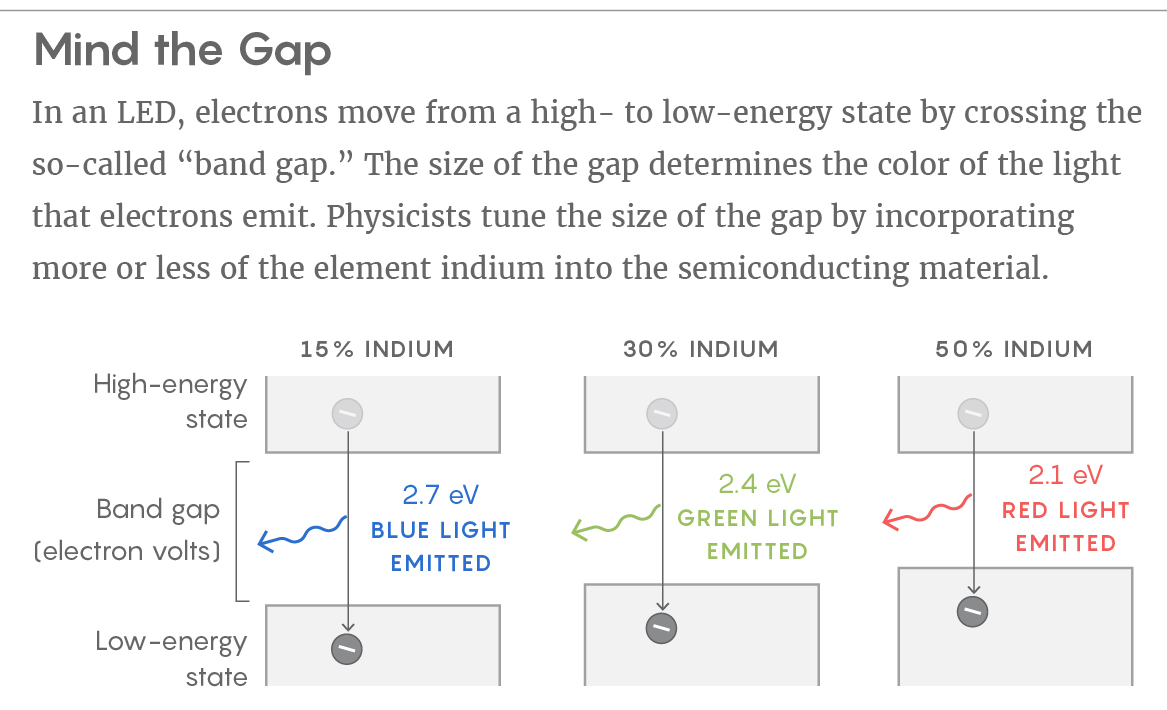
The color emitted by an LED depends on what’s called the “band gap” of the semiconducting material. The term refers to the amount of energy that electrons lose when they move from higher to lower energy states. You can think of the band gap as the “distance” the electrons move when making those jumps. The larger the distance (or gap), the bigger the difference in energy between the electron’s starting point and where it ends up — and this difference is conserved as either a phonon of heat or, preferably, a photon of light. The band gap also determines the color of the emitted light: A wider band gap leads to blue light, for example, while a narrower band gap leads to red light. (The relationship between the width of the band gap and the color of the emitted light is similar, in a sense, to the way a light wave’s frequency determines the wave’s color.)
Engineers control the band gap (that is, the color) by changing the properties of the semiconductor. In a widely used material called indium gallium nitride, LED designers tune the band gap by incorporating more or less indium into it.
Incorporating indium is a somewhat clumsy way of controlling LED color. Indium is a big atom — much bigger than the other elements in the material. When you add more of it, you push those other atoms out of position. The process generates electric fields that create more pockets of localization.
The net effect is reduced efficiency. Green LEDs have twice the indium content as blue LEDs and are half as efficient. (Red LEDs would require so much indium that physicists make them out of a different material altogether.) People in the field hope that with the landscape function, they’ll be able to design LED materials that route electrons to the light-giving positions where they’re needed most.
Lead image: Lucy Reading-Ikkanda/Quanta Magazine