Sea waves are among the world’s most misunderstood phenomena. When an incoming wave breaks on the shoreline, it appears as though the water has come to the end of a long journey, but in fact the water itself has hardly moved. Most surface sea waves transmit energy, not water, and the turbulence at the surf zone is the result of that moving energy encountering a solid obstruction—usually the shelving sea floor—against which it noisily dissipates. It is at that point that the wave transforms, from an energy-transporting wave of oscillation to a water-moving wave of translation, more commonly known as “swash.” So for most of its life a wave is not a thing so much as an event, a small part of a largescale transfer of energy from one part of the sea to another.
Waves are most commonly generated by the friction between wind and the surface of the water. As wind blows across the sea, the disturbance and perturbation cause a small wave crest to form, and the resulting up and down motion begins to transmit kinetic energy through the water in the form of a series of waves. As the waves grow, the energy (but not the water) passes from crest to crest, as is apparent when a piece of flotsam, say a tin can, can be seen bobbing up and down on the spot as waves pass beneath it.
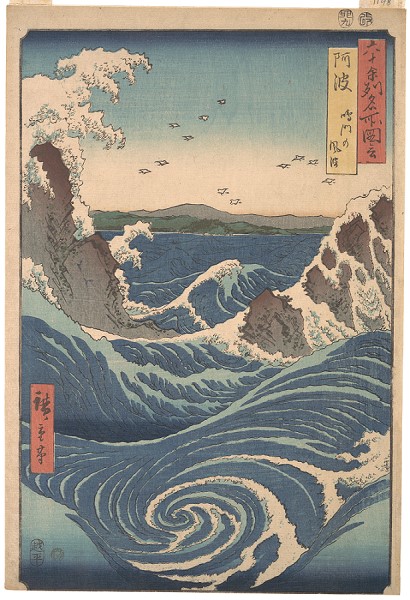
Waves are classified according to their wave period or wavelength (the distance between two crests), from the smallest capillary waves to the greatest waves of all, the tides, and those who study them are known as kumatologists, from the Greek kumas (wave), a term coined by the wave-obsessed English geographer Vaughan Cornish in 1899. Capillary waves are the small, rippled disturbances that first appear on the surface of wind-blown water, which have been known to mariners for centuries as “cats’ paws.” William Henry Smyth, in his Sailor’s Word-Book (1867), defined the cat’s paw as “a light air perceived at a distance in a calm, by the impressions made on the surface of the sea, which it sweeps very gently,” and noted the widespread superstition of rubbing a ship’s backstay to invoke the lucky cat’s paw, “the general forerunner of the steadier breeze.” Samuel Taylor Coleridge, en route to Malta in April 1804, wrote a finely observed description of the various wave types seen from deck, starting with the hair’s-breadth ripples of capillary waves:
“I particularly watched the beautiful Surface of the Sea in this gentle Breeze! – every form so transitory, so for the instant, & yet for that instant so substantial in all its sharp lines, steep surfaces, & hair-deep indentures, just as if it were cut glass, glass cut into ten thousand varieties / & then the network of the wavelets, & the rude circle hole network of the Foam.”
As is evident from both Smyth’s and Coleridge’s accounts, the characteristically rippled structure of capillary waves is due to light breezes (blowing at speeds of about 10–13 feet per second) that generate wavelengths typically less than 0.6 inch. Such gentle wind is not what sailors need to fill their sails, however, and neither is it enough to cause travelling waves to form. The threshold wavelength at which surface waves begin to travel is above 0.7 inch: anything shorter than that will be suppressed by gravity. But if—as Smyth’s sailors would have hoped—the wind strengthens to blow consistently over a substantial fetch of water, the second class of sea wave, gravity waves, will then begin to form
For most of its life a wave is not a thing so much as an event, a small part of a largescale transfer of energy from one part of the sea to another.
Gravity waves occur when wavelength grows to around five feet, and gravity joins forces with wind as the main dispersing agent. A slight convexity in the wave shape is needed to give the wind something to work on, and as soon as a wavelet develops a leeward face and a windward back, the wave (as it now is) will begin to climb: the water’s line of least resistance is to go upwards as the energy in the wind is transferred to the sea. When longer gravity waves propagate over deep water, they move rapidly away from the generating wind, at which point they are known as swells, with a typical wavelength greater than 855 feet, up to a maximum of 2,950 feet. Swells lose so little energy as they cross open water that it’s possible for one generated in the Antarctic Ocean to travel all the way to Alaska at full strength, taking several days to do so. Swells tend to flatten out as they move away from their source, and ripples and waves can form on top of underlying swells, causing a complex surface pattern which takes an experienced navigator to discern. But even the longest-lasting swell will eventually approach a shoreline, at which point contact with the shoaling sea floor will cause it to break and dissipate its energy remarkably quickly.
The Moon’s Cosmic Leash
The longest waves of all are the tides, the vertical movements of seawater dragged by the gravitational pull of the moon and sun. High tides and low tides occur on opposite sides of our planet at the same time, and, in the words of navigator Tristan Gooley, “can be thought of as a pair of very long waves that ride around the Earth,” straddling half the globe between crests. Most coastal areas experience two high tides and two low tides every lunar day—the time taken for a specific spot to rotate from and return to an exact point under the Moon: a lunar day is fifty minutes longer than a solar day because the Moon revolves around Earth in the same direction that Earth rotates around its axis, so it takes Earth a little more time to “catch up” with the Moon. The section of ocean nearest the Moon is pulled by gravity into an upward bulge, while the Earth’s rotation sends that bulge moving round the planet, giving us two high and two low tides every 24 hours and 50 minutes.
Tides are global forces, but they also feature local and regional improvisations—eddies, whirlpools, vortices, maelstroms—their cosmic regularity perturbed by wind and weather conditions, as well as by local topography. Gravitational lift alone can only raise a tide of some 18 inches: anything higher than that is caused by the shape of the coastal land and its influence on the high-tide bulge, which can be funneled into heights as great as 50 feet, as seen in the steeply shoaling Severn Estuary. When a tide makes contact with the coast, its water rushes up inlets and estuaries, reflects off sea walls, swirls around rocks and islands. It can even bounce back and cancel itself out, as happens in parts of the Gulf of Mexico, where there is only one high tide per day, while the opposite effect can lead to so-called “double tides,” where the topography and resulting flow leads to a double peak at high tide—an extra-long period of high water—which is the main reason why Southampton, shielded by the Isle of Wight on England’s south coast, was developed as a major naval and commercial port.
But beneath their capriciousness, tides express an underlying order that is almost clock-like in its regularity, with a twelve-and-a-half-hour tidal cycle governing most of our planet’s coastal waters: tides typically run for around six hours in one direction, before reversing and running in the opposite direction for the following six hours. The writer Hugh Aldersey-Williams has described this as “one of the earth’s fundamental units of time,” a temporal precondition that has made itself at home in our language, with its ancient interplays of time and tide.
Tides express an underlying order that is almost clock-like in its
regularity, with a twelve-and-a-half-hour tidal cycle governing most of our planet’s coastal
waters.
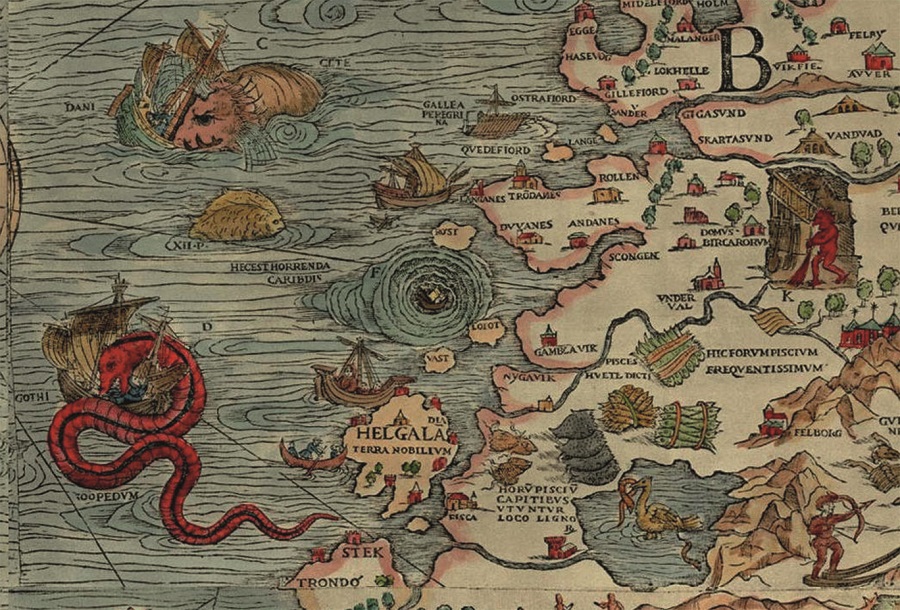
Understanding tides was vital for seafarers, and the first predictive tide table appeared in eleventh-century China in response to the powerful and deadly tidal bore that surges up the Qiantang River. Known locally as the Black Dragon, the world’s largest tidal wave attracts thousands of spectators, but the tide tables outlining wave times and heights can be notoriously inaccurate. In August 2013, the bore was roughly twice its predicted height, sweeping hundreds of spectators into the water, while the following year the appearance of a supermoon generated another unexpectedly powerful 30-foot wave that injured dozens more.
Further out at sea, tide-related perturbations such as whirlpools, vortexes, and tidal races are not always marked on charts. “They are places, but they are also theatrical events,” as Hugh Aldersey-Williams observed in the course of an account of his journey into the maelstrom, a large whirlpool off Norway’s Lofoten Islands, known locally as the Moskstraumen, after the nearby Moskenesøya Island. Rather than the wild vortex of literary legend, as invoked by Edgar Allan Poe in his celebrated story A Descent into the Maelström (1841), as well as by Jules Verne in the tragic finale of Twenty Thousand Leagues Under the Seas, the phenomenon is a system of tidal eddies and whirlpools that exude, in Aldersey-Williams’s words, “a quiet venomous authority in contrast to the petulant rage of great waves.”
Though whirlpools can be noisy and violent—they are caused when fast-moving tidal currents are sent into a spin by local topography, or by the meeting of a pair of opposing currents— the original, Norwegian, maelstrom is fairly pacific. It features a sequence of choppy waves and declivities fueled by strong diurnal tides rather than the hole in the sea—”the navel of the ocean”—implied by the popular legends.28
But Earth will not experience such phenomena forever. A billion or so years ago, the Moon was much nearer than it is today, with the correspondingly stronger gravitational attraction raising vast tides in Earth’s young oceans that had condensed from atmospheric water vapor. The energy dissipated by those tides as friction helped slow Earth’s rotational and orbital speeds, locking our Moon into its present alignment, so it always shows the same face to Earth. Eventually our slowing planet will also become tidally locked, and so always show the same face to the Moon. The tides will then cease, depriving any surviving intelligent life of one of Earth’s great natural dramas, the spectacle of the oceans’ rise and fall under the pull of the Moon’s cosmic leash.
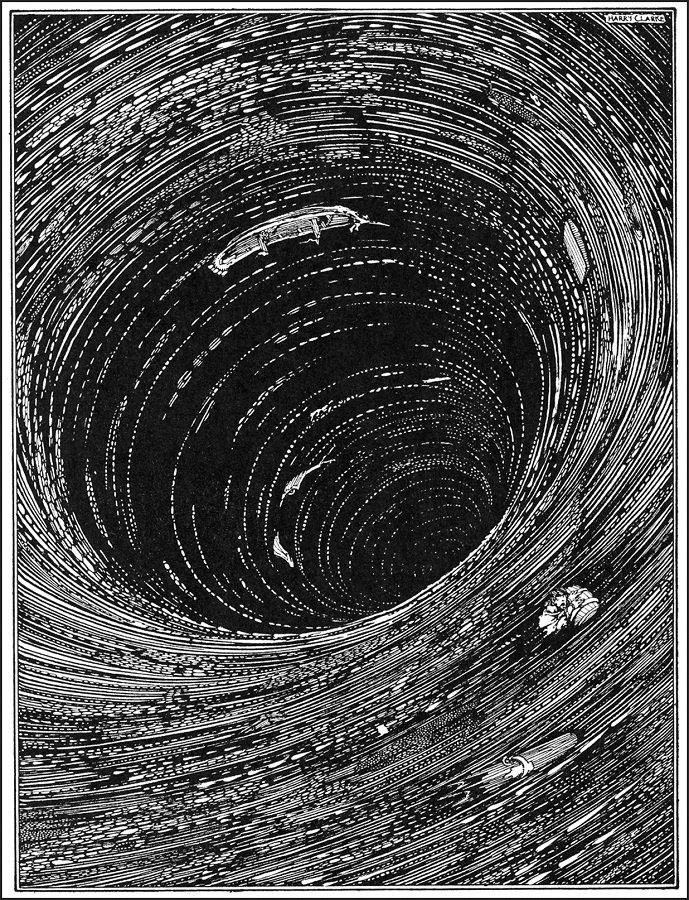
The Gulf Stream and Modern Oceanography
Among the many irritations suffered by the British during their administration of the American colonies was the fact that ships dispatched from Britain took two weeks longer to cross the Atlantic than ships dispatched from America. At a loss to account for this disparity, which could not be attributed to prevailing winds, the Admiralty eventually approached Benjamin Franklin, scientist and Postmaster General to the colonies, whose subsequent enquiries among his seafaring contacts revealed what Nantucket whalers had known for generations: the existence of a swift Atlantic current that plies its way along the eastern seaboard before heading across the open sea to Europe. Franklin, whose next two crossings were spent testing the waters, confirmed that a fast-flowing ocean river, known as the Gulf Stream, did indeed run across the North Atlantic, and that “a stranger may know when he is in it, by the warmth of the water, which is much greater than that of the water on each side of it.” It was during an Atlantic crossing in April–May 1775 that he devoted most time to the subject, lowering his thermometer into the ocean several times a day, from seven in the morning to eleven at night, while the ship kept along the eastern edge of the stream and then cut across it into colder water. He noticed that the water in the stream had a different color from the surrounding seawater, partly due to the increased quantity of gulf weed to be found in it, and that “it does not sparkle in the night.” But it was his insight that the Gulf Stream “might be studied like a river” that transformed contemporary understanding of ocean currents, while pointing out the means by which vessels, armed only with a thermometer, could shorten their crossings in both directions by keeping variously in and out of this flowing Atlantic river.
Research into the movements of the Gulf Stream was continued by Alexander Dallas Bache, a superintendent of the United States Coast Survey, who also happened to be Franklin’s great-grandson. In 1845 Bache issued a set of instructions for Gulf Stream studies that did much to establish the framework of the modern oceanographic survey:
First. What are the limits of the Gulf Stream on this part of the coast of the United States, at the surface and below the surface?
Second. Are they constant or variable, do they change with the season, with the prevalent and different winds; what is the effect of greater or less quantities of ice in the vicinity?
Third. How may they best be recognized, by the temperature at the surface or below the surface, by soundings, by the character of the bottom, by peculiar forms of vegetable or animal life, by meteorology, by the saltness of the water?
Fourth. What are the directions and velocities of the currents in this Stream and adjacent to it at the surface, below the surface, and to what variations are they subject?
The effect of the Gulf Stream’s great circulation is to transfer significant amounts of heat across the North Atlantic—a thermal budget almost one hundred times greater than world energy demand.
By bringing together a range of physical, chemical, geological, biological, and meteorological questions, Bache extended oceanography toward an understanding of the ocean as a complex system of physical and biological interrelationships. Bache was proud to have continued the oceanographic work begun by his great-grandfather, although it would cost the life of his brother, George, a naval officer in command of the survey brig Washington, who was swept overboard along with ten of his crew during a storm off Cape Hatteras while engaged in Gulf Stream research in September 1846.
In the centuries since, the course and pace of the Gulf Stream have been tracked and measured, as have the changes that it undergoes during its long Atlantic crossing: as the current heads north, its cargo of warm tropical water cools and evaporates, leaving saltier, heavier water behind. By the time it reaches the Norwegian Sea the Gulf Stream’s payload is cold and dense enough to start sinking towards the ocean floor, turning to flow south towards the equator in a cold countercurrent that will eventually return it to the point where its journey began. The effect of this great circulation is to transfer significant amounts of heat across the North Atlantic—a thermal budget almost one hundred times greater than world energy demand—preserving the inhabitants of western Europe from the harsh winters which besiege other places at similar latitudes, such as Canada or southern Alaska.
The process of thermohaline warming was first described in the 1850s by the American hydrographer Matthew Fontaine Maury, who characterized the oceans as a vast and efficient boiler house (the term derives from thermo (heat) and haline (salt), the two factors that influence the density of seawater). As Maury outlined in his landmark study The Physical Geography of the Sea (1855), “the furnace is the torrid zone, the Mexican Gulf and Caribbean Sea are the caldrons; the Gulf Stream is the conducting pipe” distributing tropical warmth towards Britain and western Europe. “It is the influence of this stream upon climate that makes Erin the “Emerald Isle of the Sea,” and that clothes the shores of Albion in evergreen robes, while in the same latitude, on this side, the coasts of Labrador are fast bound in fetters of ice,” he wrote: “There is a river in the ocean; in the severest droughts it never fails, and in the mightiest floods it never overflows. Its banks and bottoms are of cold water, while its current is of warm. The Gulf of Mexico is its fountain, and its mouth is in the Arctic Seas. It is the Gulf Stream. There is in the world no other such majestic flow of waters.”
Maury’s rhapsodic description captures the vastness of the Gulf Stream phenomenon, while hinting at its widespread influence on Earth’s weather and climate. Such thermohaline currents are the engines of Earth’s weather, due to seawater’s efficiency at storing and transporting solar heat. Water covers 70 per cent of the planet’s total area, with around 97 per cent of that water contained in the oceans; most of the remainder is locked up in ice sheets and glaciers, and less than 0.001 per cent is ever present in the atmosphere: enough for only ten days’ rain. Thermohaline circulation transports a massive current of water around the globe, from northern oceans to southern oceans and back again. These currents—driven by differences in water density—slowly turn seawater over in the ocean, from top to bottom, like a vast conveyor belt, sending warm surface water downwards and forcing cold, dense, nutrient-rich water upwards. Cold seawater in the polar regions forms sea ice, as a consequence of which the surrounding water becomes saltier, since when sea ice forms, the salt is left behind. As the polar water grows saltier, its density increases, and it starts to sink. Surface water is then pulled in to replace the sinking water, which in turn grows cold and salty enough to sink, too. This movement is what initiates the deep-ocean currents that drive the global conveyer belt, with water always seeking an equilibrium; when cold, dense water sinks, sending warmer water welling up from below to balance out the loss at the surface.
This results in an intermixing of the solar energy collected by the top layer of ocean and the nutrient-filled sediment of decayed plant and animal matter found at the bottom. Without the great conveyor belt stirring them up, along with the wind-driven upwelling that occurs near coastal regions, most of these nutrients would remain sequestered at the sea floor, leaving the oceans unable to support its dazzling array of life forms.
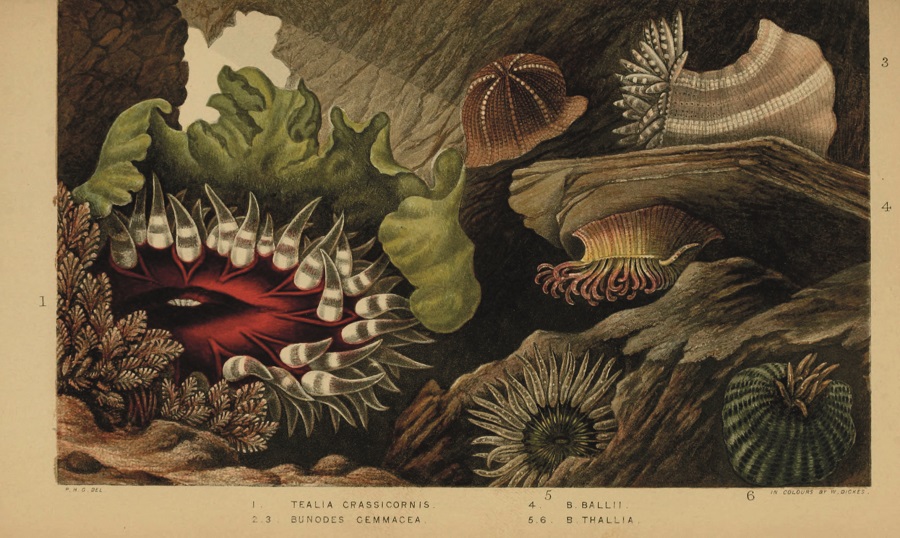
Reprinted with permission from The Sea: Nature and Culture by Richard Hamblyn, published by Reaktion Books Ltd. Copyright © Richard Hamblyn 2021. All rights reserved.
Lead image: Unusual wave refraction patterns caused by swells encountering a large merchant vessel off the coast of St Croix, United States Virgin Islands. Credit: National Oceanic and Atmospheric Administration