Every time Jim meets a patient, he cries,” Padmanee said to The New York Times in 2016. “Well not every time,” Jim added. Jim Allison and Padmanee Sharma work together at the MD Anderson Cancer Center in Houston, Texas, having met in 2005 and married in 2014. A decade before they met, Allison and his lab team made a seminal discovery that led to a revolution in cancer medicine. The hype is deserved; cancer physicians agree that Allison’s idea is a game-changer, and it now sits alongside surgery, radiation, and chemotherapy as a mainstream option for the treatment of some types of cancer.
Take one example. In 2004, 22-year-old Sharon Belvin was diagnosed with stage IV melanoma—skin cancer that had already spread to her lungs—and was given a 50/50 chance of surviving the next six months. Chemotherapy didn’t work for her and her prospects looked bleak. “I’ve never felt that kind of pain,” she later recalled, “ … you are lost, I mean you’re lost, you’re absolutely out of control, lost.” All other options exhausted, she signed up to an experimental clinical trial testing a new drug based on Allison’s idea. After just four injections over three months the tumor in her left lung shrunk by over 60 percent. Over the next few months, her tumors kept shrinking and eventually, after two and a half years of living with an intense fear of dying, she was told that she was in remission—her cancer could no longer be detected. The treatment doesn’t work for everyone but, Allison says, “We’re going to cure certain types of cancers. We’ve got a shot at it now.”
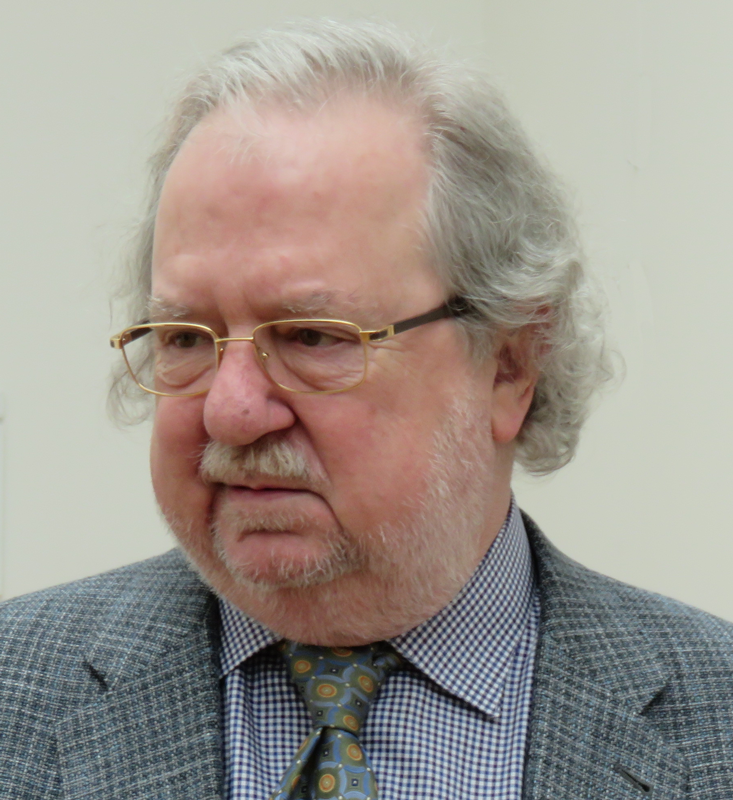
Once she had recovered, Sharon Belvin was the first patient Allison met. Her parents and husband were also there—and everybody was crying. Belvin hugged Allison tight. “There’s no words to describe what it feels like,” she has said, “ … what it feels like when you have handed someone back their life.” Both the Talmud and the Koran teach that when a person saves a life, it is as if they have saved a whole world. About two years after they met, Belvin sent Allison a photo of her first baby, and a couple of years after that, she sent him a photo of her second.
Not just a one-off success, this major new medicine has saved or prolonged thousands of lives. But it came about not from any attempt to treat a particular type of cancer, or any disease for that matter. Rather, we owe its existence to curiosity-driven research—a tinkering with cells and molecules—to fathom how the immune system works, and we’re only just beginning to understand its potential benefits.
Cancer was once thought to be invisible to our body’s defenses. As cancer is rarely caused by a germ and is rather an abnormal expansion of our body’s own cells, there tends not to be anything as obvious as a molecule from a virus, bacteria, or fungus to mark out a cell as cancerous, and for a long time a view was widely held that cancer displayed nothing to the immune system that it could recognize as alien. As long ago as 1943, the first evidence that the immune system can respond to cancer (the type not caused by a virus) was published,1 but the idea remained controversial for over three decades. This was because of the possibility that the immune reactions observed in the experiments in question had been caused not by the tumors in the animal subjects but by the chemicals used to induce the tumors. Eventually, several lines of evidence established that our immune system can and does fight cancer: immune cells were found to infiltrate tumors, and when isolated in the lab, these cells could kill tumor cells. In addition, mice engineered to lack a proper immune system were found to be especially susceptible to cancer.2
Work by Belgian scientist Thierry Boon, among others, established definitively that the genetic and epigenetic changes that turn a cell cancerous are sufficient for it to be detected by the immune system.3 Boon identified fragments of proteins that had been altered in cancer cells which can be detected by T cells (which are a type of white blood cell) as not having been in the body before. The implication of this discovery is that, as well as seeking invading germs, the immune system helps maintain the integrity of our own body’s cells, screening against detrimental genetic mutations that can arise whenever cells divide.
What CTLA-4 seemed to do was the complete opposite to what was expected.
All immune responses are multilayered and our body’s defense against cancer is no exception. As well as T cells, the white blood cells known as Natural Killer cells are also able to fight cancer. Like T cells, they do so by sending a packet of toxic proteins into a cancerous cell, but they have different strategies for detecting when a cell has turned cancerous, one of which involves recognizing protein molecules not normally found on healthy cells but which cancer cells sometimes display at their surface—so-called “stress-inducible proteins.” (Nonetheless, it is likely that cancer is recognized by our immune system less easily than is, say, the flu virus, though this is hard to prove.)
The discovery that our immune system can fight cancer led in turn to the possibility that we might tackle the disease more effectively by harnessing or boosting our immune response. In fact, this type of therapy, often called immunotherapy, has a long history. Carried out well before T cells or Natural Killer cells were even known about, a series of well-documented experiments by William Coley, performed through the 1890s, are often credited with the birth of immunotherapy. Coley, a surgeon at the Memorial Hospital, New York, noticed that a patient with neck cancer began to improve when she had a severe skin infection. He then unearthed 47 similar cases in the medical literature which propelled him to systematically test whether or not a deliberate inoculation with a mixture of heat-killed bacteria—known as “Coley’s toxins”—could help cancer patients. In an era before institutional review boards, a 29-year-old surgeon could conduct a human trial essentially on a hunch.
A century later, as we will see, Allison would need to approach human trials very differently.
While Coley’s toxins were successful in some patients, the overall effectiveness of the method was inconsistent, especially when other physicians tried to replicate it, because the concoction itself varied. The medical establishment never embraced Coley’s approach and explained away his successes as being down to initial misdiagnoses. What exactly Coley used is no longer clear, and the charity Cancer Research UK has concluded that the scientific evidence does not show that Coley’s toxins can treat or prevent cancer. Still, something of what Coley attempted lives on. The cancer researcher Steven Rosenberg found that cytokines—proteins important in cell signaling—which boost immune responses can sometimes help a person fight off cancer, although the problem with this is that cytokines switch on all kinds of processes and the storm of immune activity that follows can be toxic, occasionally lethal. So if there’s one word that encapsulates everyone’s idea as to what is most important when harnessing the immune system to fight cancer, it’s precision—in terms of selecting only those patients for treatment who are actually predisposed to respond to it (something we’ll come back to), and, most importantly for understanding Allison’s success, in terms of boosting only the precise set of immune cells that will target a patient’s cancer.
While scientists are all agreed that switching on the right set of immune cells is important, the difficulty lies in working out how to do it. One way of manipulating the immune response with precision is to use antibodies—the most precise biological agents we know. A natural part of our immune system, antibodies circulate in our blood and their job is to lock onto germs or infected cells and either incapacitate them directly or tag them for destruction. Antibodies can be produced to lock onto almost anything. Allison’s breakthrough idea was to use antibodies in a way that’s very different to how they had been used before.
His starting point was the process by which an immune response ends. When T cells initially detect an infected or cancerous cell, they multiply. Over a few days, a few hundred T cells can become many millions, expanding the small fraction of immune cells with just the right receptor to recognize the diseased cell. But an expansion of immune cells obviously cannot go on forever and after a while, in the course of a normal immune response, T cells, and other immune cells, must switch off so that the immune response winds down and the system returns to its normal resting state, usually after the threat has been cleared. Maybe, Allison thought, by stopping this “switch off” signal, immune cells could be set free to attack cancer cells more effectively, for longer. Building upon the idea that antibodies can be used to block the activity of proteins, his idea was to find a way to block the receptor protein that normally puts the brakes on an immune cell’s activity.
The reason that this was something of a paradigm shift was that while others had sought a way to turn on an immune response against cancer, Allison’s idea was to switch something off: “To unleash, not harness … the anti-tumour response,” as he puts it. The great advantage of this approach is its precision: Only those cells that have been switched on to attack the tumor would in turn have their brakes put on, so only these cells, not every immune cell in the body, would be unleashed by the intervention. This approach has become known as immune checkpoint therapy.
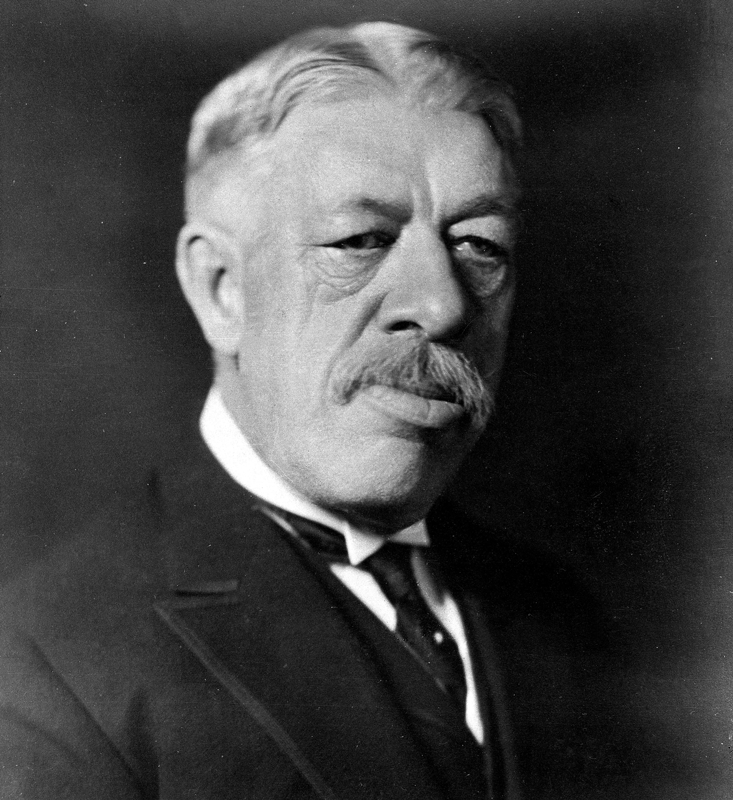
Allison never set out to study cancer—“That wasn’t it at all,” he says—he set out to understand how T cells work. But cancer was on his mind because he had lost his mother, two uncles, and then later his brother, to cancer, and had seen firsthand the ravaging side effects that radiation and chemotherapy can have. He had graduated from school early, started college at age 16 and knew already then that he wanted to be a scientist. At that time, T cells had just been discovered as a specific type of white blood cell. It was by studying the role of various receptor proteins on the surface of T cells that Allison, along with others, stumbled upon the T cell’s brake system.
It’s often said that a journey of a thousand miles begins with a single step. But pinpointing that first step in the journey toward any scientific idea is especially hard because every new idea is built upon the ideas that preceded it. Allison’s discovery was no exception. The immunologist Charles Janeway realized that the presence of something that has never been in your body before could not be the sole trigger for an immune reaction; a second signal must be needed. For Janeway this was the detection of germs; for the immunologist Polly Matzinger, it was the detection of something dangerous. The Nobel Laureate Ralph Steinman discovered that dendritic cells are particularly good at detecting germs. When they do so, they signal to T cells that a germ is present—providing the second signal needed to kick-start an immune reaction—by displaying so-called co-stimulatory proteins at their surface. These proteins on the dendritic cells fit into receptor proteins on the surface of T cells like a key in a lock, essentially unlocking the T cell’s potential. It’s here that Allison’s journey can be said to have begun: with the identification of a second receptor protein on the surface of T cells that was uncannily similar—about 30 percent identical—to the one “unlocked” by co-stimulatory proteins, but whose role in our immune system remained a mystery.
The mysterious receptor had been given an especially cumbersome name: cytotoxic T-lymphocyte-associated molecule 4, or CTLA-4, named for simply being the fourth in a series of molecules identified on T cells. (It is also the registration number of Allison’s Porsche. Presumably there’s far less of a demand for the number plate “CTLA4” than there is for, say, his first name “Jim.”) Not that Allison discovered CTLA-4 himself: It was identified in 1987 in Pierre Golstein’s lab in Marseilles, as part of his lab’s mission at that time to discover which genes are active exclusively in T cells and no other white blood cells. Golstein didn’t pursue his discovery of CTLA-4 and uncover its role. He simply showed that it was present at the surface of T cells switched on to participate in an immune response—whereas it was not present on T cells that were resting, simply waiting for signs of trouble. This indicated that the molecule was somehow important only once an immune response had got going. Not much to go on, but intriguing.
Switching off an immune response is just as vital to health as switching it on.
The results of experiments aimed at revealing what CTLA-4 does in the body were, at first, interpreted in line with expectation: that the protein receptor helped stimulate T cells. It was, after all, very similar to another stimulatory receptor, and redundancy of this sort is built into the immune system, with many different molecules and cells having overlapping tasks, presumably to help the system be robust: Such redundancies ensure that if a germ were to interfere with any one component, there’s a good chance that its function can anyway be performed by another component. But in 1994, Jeff Bluestone and his research team at the University of Chicago—urged on by Bruce Springsteen’s music, which was playing in the lab—stumbled across the fact that what CTLA-4 seemed to do was the complete opposite to what was expected.
At the time, Bluestone’s team had produced an antibody to block the CTLA-4 receptor, which allowed them to test what would happen to T cells if CTLA-4 was incapacitated. His lab’s overarching mission was to find ways of stopping an immune response in order to help address the problems of organ transplantation or autoimmune disease. Like everyone else, Bluestone presumed that CTLA-4 was likely to be a stimulatory receptor—an on-signal—and that by blocking it, the immune system would be rendered less effective.
He says he’ll never forget the day when student Teresa Walunas came into his office and showed him the results: that blocking CTLA-4 with an antibody caused T cells to react more, not less.4 If blocking CTLA-4 led to a stronger reaction, then CTLA-4 must normally deliver an off-signal, not a stimulatory signal. Because the result went so directly against the prevailing view, finding it didn’t feel like a Eureka moment. As Bluestone recalls, his reaction was more like: “Gee, maybe it’s an off-molecule but boy it will be hard to prove that to anybody.”
Two of Bluestone’s friends had recently helped set up a new scientific journal called Immunity, so he published his discovery there. At the time, Bluestone was worried that his work might be hard for others to find if the new journal didn’t take off, but he needn’t have been concerned. It soon became one of the world’s top journals for research about the immune system.
Allison, then director of the Berkeley Cancer Research Laboratory at the University of California—and Bluestone’s rival—had, in 1989, charged his own Ph.D. student Matthew “Max” Krummel with the very same problem: finding out what CTLA-4 does. Allison didn’t have a specific idea he wanted to test; it was curiosity-driven rather than hypothesis-driven science, as Krummel puts it. Initially unaware of what Bluestone’s lab were up to, Krummel also made antibodies that could lock onto CTLA-4 so he too could test what happens to an immune response when CTLA-4 is blocked. Making an antibody wasn’t so easy at that time—it is much easier nowadays—and it took Krummel four years to hit upon a recipe that worked. Once he had the antibody in hand, his experiments using it gave the same result as Bluestone’s: Blocking CTLA-4 could boost an immune reaction, consistent with the idea that CTLA-4 normally delivers a switch-off signal to T cells.5
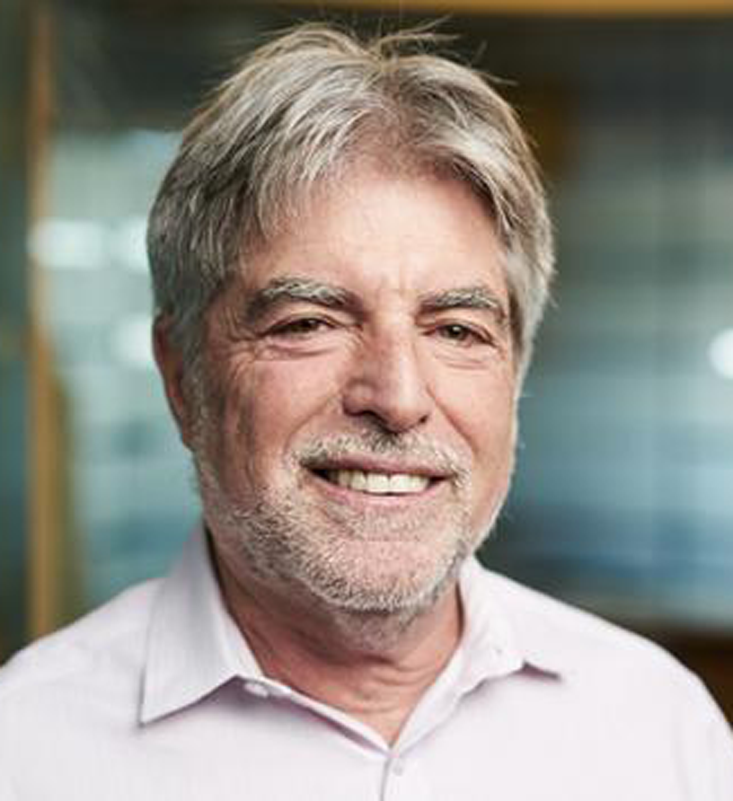
Even though both Bluestone’s and Allison’s labs came to this same conclusion, their discovery was still controversial. In part, this was because an antibody stuck to CTLA-4 may block the receptor from working, but it may also, in principle, do the opposite and trigger it, and blocking a switch-off signal would provide the same result as triggering a stimulatory signal. The controversy was resolved when mice genetically altered to lack CTLA-4 were found to die at a young age because of a massive expansion of immune cells, overrunning the animal’s body, producing toxic levels of inflammation.6,7 This plainly showed that CTLA-4 was vitally important for switching off an immune response—and also established that switching off an immune response is just as vital to health as switching it on.
Next, Krummel produced large amounts of his antibody in order to test the effect of blocking CTLA-4 on different types of immune response. But the particular experiment which proved decisive and led to a medical breakthrough was one that Krummel didn’t have time to do himself. Krummel was busy testing how blocking CTLA-4 affected immune responses against proteins from bacteria, so Allison asked a new arrival in his lab, Dana Leach, to test how blocking CTLA-4 affected the immune response to tumors.
Leach injected the antibody into mice with bowel cancer. Allison hoped that by blocking the T cell switch-off signal, tumors in the colon might be attacked more effectively by the immune system and their growth might slow down. The results were even better than he had hoped. “When Dana Leach … showed me the initial data, I was shocked and surprised,” Allison later recalled. In all the animals treated, the tumors had regressed completely.8 Over the Christmas holiday of 1994, they repeated the experiment blind, so that the person analyzing the mice wouldn’t know which animals had been given the treatment. Leach set up the experiment, went off to see his girlfriend for the holiday season, and Allison himself measured the tumors. At first, the tumors stayed the same size. Then, after two weeks—“as if by magic”—the tumors began to regress in just one group of mice. A little later, they had completely disappeared. That group of mice was, of course, the one that had received the treatment. ‘The fact that blockade of a single molecule could lead to complete tumor regression was astonishing,’ Allison said.
A lot of deal-making takes place alongside the science when a new cancer drug is at stake.
Over the next 15 years, Allison’s team, and others, found that blocking CTLA-4 could help treat many different types of cancer in mice. Good news for mice but the next step was to test this in humans. In hindsight it is hard to believe, but Allison met with great resistance when he approached companies and funding agencies to do this. At the time many physicians, academics, and industry scientists were profoundly skeptical about harnessing the immune system to fight cancer because so many previous attempts, using cytokines or dendritic-cell vaccines for example, had largely failed and led to complicated side effects. “Some of my friends, very renowned,” Allison recalls, “ they wanted to insult me in public, they’d say Jim’s a tumor immunologist, [and] snigger.”
It took around two years before Allison could get a company interested in his idea. Eventually, immunologist Alan Korman at Nexstar, a biotech company in Colorado, began working on an antibody to block human CTLA-4, after licensing the idea from Allison’s university. Nexstar sublicensed the idea to another company, Medarex, based in New Jersey, who had recently acquired a third company, GenPharm, which specialized in producing antibodies that could be safely used in humans. All this led to an antibody— named MDX-010—which Allison and others could use in clinical trials. The process couldn’t have been any further from William Coley’s in the 1890s, who simply tested his idea on patients without delay.
In the first small trials, MDX-010 gave a durable response in a fraction of patients but led to adverse side effects in other patients. Larger trials gave mixed results. What helped save the drug from being dropped was that it performed much better when the criteria by which a cancer treatment was deemed successful were changed. That’s not to say the rules were twisted simply to make the drug look better. Rather, astute clinicians realized that in some cases, according to the existing rules, this new medicine would be regarded as a failure even when patients actually benefited.
The reason was that success for cancer drugs had been defined with chemotherapy in mind. These agents often kill cancer cells directly and if the treatment is successful, a person’s tumor can become smaller within weeks. For trials using the antibody to block CTLA-4—unleashing the power of the immune system—little might happen at first. Measurements of the tumor would sometimes show that its size increased, formally indicating that the treatment had failed. But these numbers lied. Later, presumably after the immune system had been given enough time to get going, the tumor might then shrink. We now know that a tumor might initially get bigger after treatment—seemingly bad news for the patient—because immune cells move into the tumor causing it to swell—which is actually good news for the patient.9
For medicines which harness the immune system, the World Health Organization’s criteria for success in a cancer trial had to be changed. These rules—now known as the Immune-Related Response Criteria—included an increase in the time allowed for the treatment to work. An initial increase in tumor size was recognized as a tumor flare, and not necessarily a sign that the treatment had failed. These changes transformed the fate of what would become a life-saving cancer drug and shows why the relationship between the pharmaceutical industry and regulators is complicated; they must work together, as well as independently, to scrutinize new medicines.
When their interim data suggested that using their antibody to block CTLA-4 was not superior to chemotherapy, the pharmaceutical giant Pfizer dropped the idea, but Medarex persevered. Pfizer may have just made the decision too soon; an improvement in the overall survival of patients might have become apparent later. At any rate, Pfizer sold the rights for their antibody to another company, MedImmune, owned by AstraZeneca. Once the success of this type of therapy became clear, Pfizer changed its mind and agreed to pay up to $250 million to a small biotech company in 2016 in order to once again have proprietorship of an antibody able to block CTLA- 4. Medarex, on the other hand, were rewarded for their perseverance. In 2009, New York-based Bristol-Myers Squibb bought Medarex for over $2 billion, primarily for their antibody that blocked CTLA-4. At the time, the antibody was still being assessed in clinical trials; it hadn’t been proven to work but was evidently worth a $2 billion bet. Needless to say, a lot of deal-making takes place alongside the science when a new cancer drug is at stake.
Shortly after Medarex was acquired by Bristol-Myers Squibb, blocking CTLA-4 was proven to work, thanks to the new Immune-Related Response Criteria. In a decisive trial of melanoma patients, overall survival was used as the principal criteria for evaluation rather than other measures of a response such as a change in tumor size. For all the patients in this trial, their cancer had spread from the skin to other places in the body and life expectancy was short. The results, announced on June 5, 2010 to over 30,000 delegates at an annual cancer meeting in Chicago and simultaneously published in the prestigious New England Journal of Medicine,10 showed that the average survival of patients went up from about six to 10 months when they were treated with the antibody that blocked CTLA-4. This was an unprecedented result: No previous clinical trial had revealed anything to be capable of increasing the average lifespan of such late-stage melanoma patients. Even more amazing was that some patients enjoyed a long-term benefit. Over 20 percent lived another two years or more. Some of those who received the drug early on have since survived more than 10 years.11 Sharon Belvin is one.
In March 2011, the U.S. Food and Drug Administration approved the new medicine, by which time it had been given a generic drug name ipilimumab (not necessarily a huge improvement on MDX-010) and the brand name Yervoy. Immediately, it was predicted that 15 percent of the 68,000 patients diagnosed with melanoma every year in the U.S. would receive Yervoy. With a course of four doses initially costing upward of $80,000, worldwide sales of the drug were predicted to reach $2 billion in 2015. The hype turns out to have been almost justified: Actual sales of Yervoy in 2015 were $1.1 billion. Not surprisingly, the development of medicines to harness the immune system has become the fastest-growing area of the entire pharmaceutical industry.
Daniel M. Davis is professor of immunology at the University of Manchester in the U.K. He is the author of The Compatibility Gene: How Our Bodies Fight Disease, Attract Others, and Define Our Selves.
Reprinted with permission from The Beautiful Cure: The Revolution in Immunology and What It Means for Your Health by Daniel M. Davis, published by The University of Chicago Press. © 2018 by Daniel M. Davis. All rights reserved.
References
1. Gross, L., Intradermal immunization of C3H mice against a sarcoma that originated in an animal of the same line. Cancer Research 3, 326–333 (1943).
2. Shankaran, V., et al. IFNgamma and lymphocytes prevent primary tumour development and shape tumour immunogenicity. Nature 410, 1107–1111 (2001).
3. Coulie, P.G., Van den Eynde, B.J., van der Bruggen, P., & Boon, T. Tumour antigens recognized by T lymphocytes: at the core of cancer immunotherapy. Nature Reviews Cancer 14, 135–146 (2014).
4. Walunas, T.L., et al. CTLA-4 can function as a negative regulator of T cell activation. Immunity 1, 405–413 (1994).
5. Krummel, M.F. & Allison, J.P. CD28 and CTLA-4 have opposing effects on the response of T cells to stimulation. The Journal of Experimental Medicine 182, 459–465 (1995).
6. Tivol, E.A., et al. Loss of CTLA-4 leads to massive lymphoproliferation and fatal multiorgan tissue destruction, revealing a critical negative regulatory role of CTLA-4. Immunity 3, 541–547 (1995).
7. Waterhouse, P., et al. Lymphoproliferative disorders with early lethality in mice deficient in Ctla-4. Science 270, 985–988 (1995).
8. Leach, D.R., Krummel, M.F. & Allison, J.P. Enhancement of antitumor immunity by CTLA-4 blockade. Science 271, 1734–1736 (1996).
9. Hoos, A. Development of immuno-oncology drugs—from CTLA4 to PD1 to the next generations. Nature Reviews Drug Discovery 15, 235–247 (2016).
10. Hodi, F.S., et al. Improved survival with ipilimumab in patients with metastatic melanoma. New England Journal of Medicine 363, 711–723 (2010).
11. Schadendorf, D., et al. Pooled analysis of long-term survival data from phase II and phase III trials of ipilimumab in unresectable or metastatic melanoma. Journal of Clinical Oncology 33, 1889–1894 (2015).