The first pieces of the brain’s “inner GPS” started coming to light in 1970. In the laboratories of University College London, John O’Keefe and his student Jonathan Dostrovsky recorded the electrical activity of neurons in the hippocampus of freely moving rats. They found a group of neurons that increased their activity only when a rat found itself in a particular location.1 They called them “place cells.”
Building on these early findings, O’Keefe and his colleague Lynn Nadel proposed that the hippocampus contains an invariant representation of space that does not depend on mood or desire. They called this representation the “cognitive map.”2 In their view, all of the brain’s place cells together represent the entirety of an animal’s environment, and whichever place cell is active indicates its current location. In other words, the hippocampus is like a GPS. It tells you where you are on a map and that map remains the same whether you are hungry and looking for food or sleepy and looking for a bed. O’Keefe and Nadel suggested that the absolute position represented in the hippocampal place cells provides a mental framework that can be used by an animal to find its way in any situation—be that to find food or a bed.
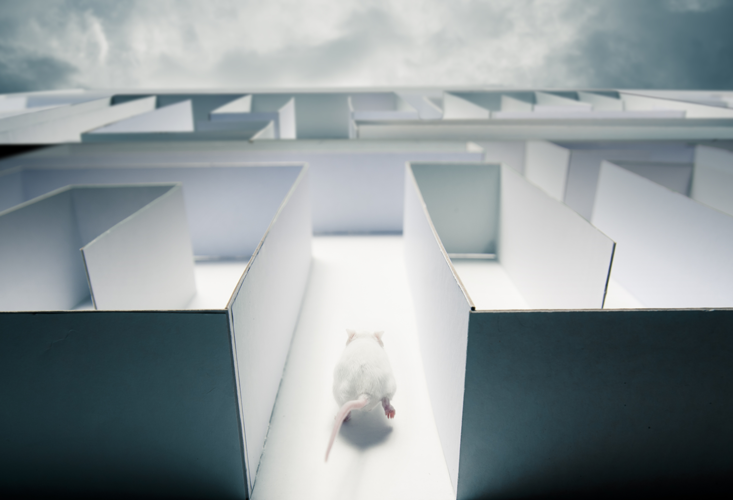
Over the next 40 years, other researchers—including the husband and wife duo of Edvard and May-Britt Moser—produced support for the idea that the brain’s hippocampal circuitry acts like an inner GPS.3 In recognition of their pioneering work, O’Keefe and the Mosers were awarded the 2014 Nobel Prize in physiology or medicine. You’d think that this would mean that the role of the hippocampus in guiding an animal through space was solved.
But studying the brain is never that straightforward. Like a match lighting a fuse, the 2014 Nobel Prize set off an explosion of experiments and ideas, some of which have pushed back against O’Keefe and Nadel’s early interpretation. This new work has suggested that when it comes to spatial navigation, the hippocampal circuit represents location information that is relative and malleable by experience rather than absolute. The study of the hippocampus seems to have stumbled into an age-old philosophical argument.
Not only is the space represented by the hippocampus not absolute, but it looks like it can be modified by observing the experiences of others.
Physics grappled with the question of whether space is absolute or relative for centuries, before deciding in favor of relativity. But, it is only in recent years that the brain sciences have begun to discuss a parallel set of questions. For many years now, absolute space has ruled neuroscience. In the visual system, for example, it has long been assumed that there are two channels of information flow.4 The first is the “what” channel, carrying information about the identity of objects that an animal sees. The second is the “where” channel, containing information about the absolute position of these objects. It was believed that the “what” channel contained no positional information at all. However, recent work has shown that while no information about the absolute position of an object is present in this channel, there is relative position information.5,6 This relative positional information is likely to be very important for object recognition.
Findings like this have provided a foothold for the idea that relative information can be important to the brain. But it has been the recent marriage of neuroscience with the fields of computer science and artificial intelligence that have really strengthened this perspective. Work at this interface has shown that a brain that uses an absolute, invariant model of the world to model and negotiate changing environments requires more computational resources than one that uses relative information. Understanding when and where our brains use relative and absolute information could provide insights into the working, flexibility, and speed of its subsystems and into our own behaviors. The hippocampus, in particular, could be one of the first waypoints in this debate.
A key challenge to O’Keefe and Nadel’s absolute-location interpretation of hippocampal place cell activity came in a paper published late last year from Kimberly Stachenfeld, Matthew Botvinick, and Samuel Gershman.7 The researchers, affiliated with Google DeepMind, Princeton University, University College London, and Harvard University, suggested that instead of representing an animal’s absolute position, the hippocampus represents where the animal is likely to go in the immediate future. This representation takes into account its preferred movements and learned habits. The hippocampus, in this view, is a predictive map and not an absolute one.
Previous studies had shown that the activity of a place cell steadily decreases as the animal moves away from the center of a given area of interest. O’Keefe and Nadel interpreted this to be a sign that the place cell represents the animal’s current location. In the framework proposed by Stachenfeld and her colleagues, the amount of activity was proposed to represent how likely it is that the animal will be in the center of the area of interest in the next instant in time. If it already is at the center, then it is highly likely that it will still be there a moment later, and so the activity of the place cell is high. If it is so far away from the center of the area that it can’t reach it in the next instant, then the place cell is not active.
O’Keefe’s and Stachenfeld’s theories may sound similar, and both seem to explain the basic properties of place cell activity. However, they make different assumptions about the nature of the spatial map in the hippocampus and it is only through clever experimental design and computational model testing that the two explanations can be distinguished. Stachenfeld, Botvinick, and Gershman did this by reanalyzing data from previously published research studies and finding that some of it could be explained by their model of hippocampus function but not the traditional model. The most striking of these examples is data from a study by Alice Alvernhe and colleagues in Marseille, France.8 These researchers used something called the Tolman detour maze, which requires the rat to run along a straight pathway from start to end. In some situations the straight path was blocked, forcing the animal to take one of two C shaped detours.
The hippocampus carries far more than just an invariant representation of one’s own position in space.
Under O’Keefe’s cognitive-map interpretation of place cell activity, a cell that was active when the rat was at the intersection of the detour and the straight path would fire in the same way regardless of whether the straight path was blocked or not. This was not what was observed: In fact, this cell behaved differently depending on whether the path was blocked or not. The rat’s previous experience affected how its place cells fired. An absolute map would not function this way. What’s more, Stachenfeld and colleagues performed computer simulations to show that the place cell activity seen in the study by Alvernhe and colleagues matched their predictive-map hypothesis much better than that of O’Keefe’s cognitive-map hypothesis.
The Stachenfeld challenge to the O’Keefe-Nadel interpretation argued that place cells don’t encode an absolute position, but one that is relative to its own position history, experience, and behavioral preferences. Just a few months later, another set of studies showed that place-cell activity is also modulated by the location of other animals of the same species.9,10,11 In papers published this year, Nachum Ulanovsky from the Weismann Institute in Israel and Shigeyoshi Fujisawa at the RIKEN Brain Science Institute in Saitama, Japan trained animals to follow a given pathway by observing training runs performed by other animals of the same species (Ulanovsky used bats and Fujisawa used rats). When the animals followed the prescribed route, place cells in their hippocampus fired, as expected. What was unexpected was that a subset of these same place cells also fired while the animals were observing the training runs. The researchers termed these neurons social place cells.
These results are again at odds with the original interpretation of place cell activity that described the place cells as representing position in an absolute space. Not only is the space represented by the hippocampus not absolute, but it looks like it can be modified by observing the experiences of others.
Place cell activity has proven to be far more complicated than previously imagined. The classical Nobel Prize-winning view of the hippocampal circuit’s role in spatial navigation has turned out not to be the whole story, and the hippocampus carries far more than just an invariant representation of one’s own position in space.
The idea that the activity of a place cell is predictive and can be modified by learning and the behavior of other animals may make it easier to conceptualize an all-encompassing framework that ties together the hippocampus’ role in spatial navigation with its well-established role in learning and memory formation. Since the discovery (in a human patient named H.M.) that hippocampal removal can lead to the inability to form new memories, the hippocampus has been studied as one of the primary sites of memory formation in the brain.12 While it has also been known since O’Keefe and Dostrovsky’s initial experiments that the hippocampus plays a basic role in spatial navigation, how and why this tiny portion of the brain can host both spatial maps and complex memories has remained poorly understood. But the emerging understanding that our spatial maps are themselves relative and affected by memory and behavior makes the dual function of the hippocampus easier to understand. Fifty years from O’Keefe and Dostrovsky’s first observations, we are beginning to get a clearer view of how this key brain area makes us who we are.
Adithya Rajagopalan is a first-year graduate student in the department of neuroscience at Johns Hopkins University.
References
1. O’Keefe, J. & Dostrovsky, J. The hippocampus as a spatial map. preliminary evidence from unit activity in the freely-moving rat. Brain Research 34, 171–175 (1971).
2. O’Keefe, J. & Nadel, L. The Hippocampus as a Cognitive Map Oxford University Press (1978).
3. Rowland, D.C., Roudi, Y., Moser, M.B., & Moser E.I. Ten years of grid cells. Annual Review of Neuroscience 8, 19-40 (2016).
4. Ungerleider, L.G. & Mishkin, M. Two cortical visual systems. In Ingle, D.J, Goodale, M.A., & Mansfield, R.J.W. (Eds.) Analysis of Visual Behaviour MIT Press, Cambridge, MA (1982).
5. Vaziri, S., Carlson, E.T., Wang, Z., & Connor, C.E. A channel for 3D environmental shape in anterior inferotemporal cortex. Neuron 84, 55–62 (2014).
6. Hong, H., Yamins, D.L.K., Majaj, N.J., & Dicarlo, J.J. Explicit information for category-orthogonal object properties increases along the ventral stream. Nature Neuroscience 19, 613–622 (2016).
7. Stachenfeld, K.L., Botvinick, M.M., & Gershman, S.J. The hippocampus as a predictive map. Nature Neuroscience 20, 1643–1653 (2017).
8. Alvernhe, A., Save, E., & Poucet, B. Local remapping of place cell firing in the Tolman detour task. European Journal of Neuroscience 33, 1696–1705 (2011).
9. Omer, D.B., Maimon, S.R., Las, L., & Ulanovsky, N. “Social place-cells in the bat hippocampus. Science 359, 218–224 (2018).
10. Danjo, T., Toyoizumi, T., & Fujisawa, S. Spatial representations of self and other in the hippocampus. Science 359, 213–218 (2018).
11. Abbot, A. ‘Bat-Nav’ Reveals How the Brain Tracks Other Animals Nature News (2018).
12. Scoville, W.B. & Milner, B. Loss of recent memory after bilateral hippocampal lesions. Journal of Neurology, Neurosurgery & Psychiatry 20, 11–21 (1957).
Lead Image Credit: Susan Law Cain / Shutterstock