Eugene Rosenberg, a coral microbiologist, ran into a rather large problem in the early 2000s. While working at the University of Tel Aviv in Israel, he discovered that he couldn’t replicate his own breakthrough findings from a decade earlier. What seemed like a potentially devastating failure at the time would lead Rosenberg to a new way of thinking about evolution.
In the 1990s, he’d discovered a driver of coral disease. Rising ocean temperatures had begun to cause coral bleaching in the Eastern Mediterranean. No one really understood why bleaching occurred, only that if a coral polyp remained without its algae for too long, it could starve and die. Some argued that polyps expelled algae because, at higher temperatures, the stressed algae stopped contributing to the symbiosis. They became, in a sense, unproductive employees, and were summarily fired.
But after a series of experiments, Rosenberg had come to a different conclusion. He’d noticed clumps of rod-shaped bacteria amassed at the borders of bleached regions of corals, suggesting infection. If he first treated the seawater with antibiotics, killing the resident bacteria, and then raised the temperature on corals in tanks, the corals never bleached. Heat alone didn’t drive disease, it seemed. Bacteria somehow contributed.
He isolated one bacterium called Vibrio shiloi—a distant relative of cholera—that prompted coral disease. It was an opportunist: harmless at normal temperatures, but pathogenic when conditions grew warmer.
In 1996, he published his findings in Nature. But now, a decade later, he and his graduate students tried to replicate the experiment, and failed—twice. The vibrio bacterium no longer seemed to cause disease.
“The coral had become resistant,” Rosenberg told me. “This was a blow.”
He began brainstorming possible explanations. Humans and other vertebrates have adaptive immune systems. With exposure to pathogens, our immune systems can learn and remember. When we encounter a pathogen we’ve seen before, we can repel it before it harms us. That’s the principle underlying vaccines. But corals lack an adaptive immune system. They couldn’t gain immunity from prior exposure the same way.
Just as infectious diseases could spread through a population, causing illness and death, so might beneficial microbes disperse quickly.
One day, during a morning walk, Rosenberg mentioned the problem to his wife, Ilana Zilber-Rosenberg. She is a microbiologist and nutritionist, and immediately had a thought: probiotics, microbes that improve health. In people, she explained, native bacteria helped protect against pathogens. That’s why people who took antibiotics could, paradoxically, develop new infections after treatment. Wiping out commensal microbes opened the door to opportunists.
Perhaps in the period between the first experiment and the last, the corals had acquired new microbes that now shielded them from V. shiloi.
Back in the lab, Eugene tested the idea. He treated the corals with antibiotics, killing off whatever microbes they harbored. Then he repeated his original experiment, exposing the corals to V. shiloi. Now, as he cranked up the heat, the corals bleached. (Some scientists dispute that an infection causes bleaching because they failed to isolate those vibrio bacteria from bleached coral. Rosenberg says the null findings are beset by methodological flaws. Other researchers have corroborated his findings, he points out.)
What was the probiotic the corals had acquired? Rosenberg isolated one bacterium, which he dubbed EM3. When introduced to corals, it conferred resistance to V. shiloi. Probably not by accident—EM3 was a vibrio species, belonging to a genus of motile, comma-shaped bacteria native to salt water. The corals had made friends with a relative of their enemy. This new alliance had helped them adapt to changing conditions.
Rosenberg realized that he’d stumbled onto an important biological principle: adaptation not by genetic mutation or recombination, but by microbial adjustment. The modification had occurred remarkably quickly, in three years, potentially within a single coral’s lifespan. His insight was that just as infectious diseases could spread like wildfire through a population, causing illness and death, so might beneficial microbes disperse quickly, boosting health and promoting survival.
“If you can have disease epidemics,” he said to me, “why not probiotic epidemics?”
Rosenberg calls the broader concept the “hologenome theory” of evolution. The term was first used by molecular biologist Richard Jefferson in 1994. (Microbes detected during genetic analysis of multicellular organisms shouldn’t be viewed as contamination, Jefferson said, but as integral to the organism studied.)
Rosenberg, who came to the term independently, argues that an organism and its associated microbes—the “holobiont”—are a single evolutionary entity. “We consider this to be a level of selection in evolution,” says Rosenberg. “I compete against you not just based on my genes, but on my bacteria.”
Some think Oculina patagonica, the coral Rosenberg studies, is an invasive species brought to the Mediterranean by ships. Others claim it’s a native that’s expanding its range because of environmental changes. Either way, its changing prevalence highlights a reality of life in what many call the Anthropocene epoch: Human activity has left no ecosystem untouched. That translates to multiple new selective pressures bearing down on life forms ranging from corals, to frogs, to us. Mass extinction is one well-founded worry. But during this period of biotic upheaval, we might also see occasional examples of rapid adaptation enabled by microbes. Conservationists in particular are closely watching these dynamics, which could represent a new leverage point in the quest to help some wildlife survive. Those who study human microbes, meanwhile, already consider them an important, and historically overlooked, factor contributing to our health and disease.
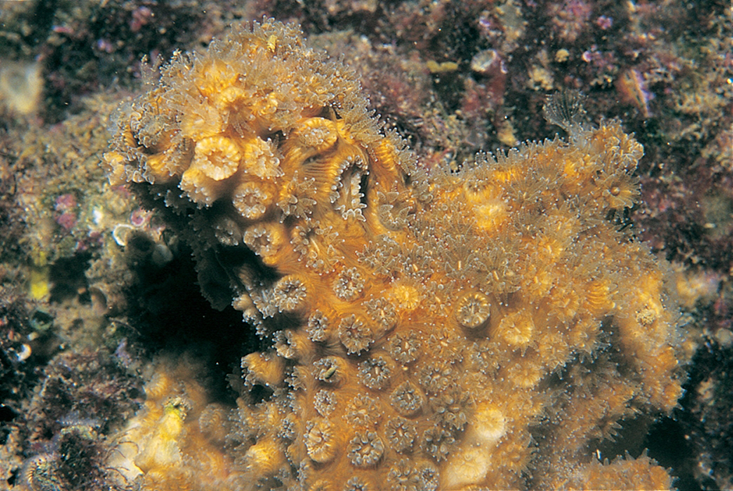
Ever since Charles Darwin taught us that life is constantly driven to change by myriad pressures—that species are not static, but are constantly morphing—biologists have debated how fast those changes can occur. The discovery of the genome a century after Darwin published On the Origin of Species seemed to demarcate an upper limit. Animals could evolve only as fast as advantageous genes could arise and spread, and as existing genomes could be reshuffled through sexual reproduction. But then came “epigenetics”: Some adaptation could occur more quickly, without changing genes themselves, but by altering how existing genes translated into living flesh.
Rosenberg and others are proposing an even quicker process—organisms can adapt rapidly by switching their symbiotic microbes. The very quality that makes pandemics so terrifying—rapid contagion—may also drive mass adaptation.
To support the hologenome theory, Rosenberg and others look to the study of the human microbiome, particularly the example of Clostridium difficile. People tend to acquire the bacterium during hospital stays and after they’ve “clearcut” their native microbes with antibiotics directed at another infection. C. difficile then blooms. Patients can end up delirious and in pain, weakened by an unremitting torrent of bloody diarrhea. The microbe infects an estimated 500,000 Americans yearly, killing some 29,000 of them within a month.
C. difficile is increasingly resistant to antibiotics, so further treatment fails in about 1 in 5 cases. For this subset, the “fecal transplant” is nearly miraculous. The procedure involves “implanting” stool from a healthy person, via enema, or in pills, into the intestines of one stricken with C. difficile. And it’s 94 percent effective at curing C. difficile. It works, scientists think, by restoring a fully functioning microbial ecosystem in one fell swoop. The donor’s tight-knit community deprives the pathogen C. difficile of an ecological niche, squeezing it from the gut.
Technically, stool transplants are not “probiotic epidemics.” But the principle the transplant illustrates—that native microbes prevent disease, and that tweaking those microbes can fend off a deadly infection—is precisely what scientists think could drive a probiotic epidemic.
The amphibious fungus has occasioned one of the worst die-offs of wildlife: 200 are extinct; 500 more are infected.
How animals, from corals to humans, acquire and cultivate their microbial communities remains mysterious. In some animals, microbes may come from parents, peers, and the greater environment. Different diets also affect the microbiota, indicating some flexibility in the microbes a species can harbor. And then there’s mucus itself, which repels some microbes while selectively feeding others.
Unlike mammals, whose mucous membranes are mostly internal, corals wear their mucus on both the inside and the outside. But the same principle may apply. Researchers, including Max Teplitski, a scientist at the University of Florida, Gainesville, and his colleagues find that coral mucus seems to attract and cultivate a particular community of microbes. The pathogenic vibrio may be present, but at normal temperatures other microbes keep it in check. When temperatures rise, however, the vibrio begins reproducing rapidly, overwhelming the protective commensals. That’s when coral disease occurs.
Teplitski, who has studied these dynamics in Caribbean Elkhorn coral, describes the result as “dysbiosis”: an imbalance of always-present microbes. Pimples, yeast infections, and dental cavities are examples of dysbiotic diseases in people—conditions caused not necessarily by newly arrived pathogens, but by ever-present microbes that bloom and harm their host.
The mystery of Rosenberg’s corals is how they acquired new microbes able to protect them at higher temperatures. In corals there’s some evidence of a deliberate process—not with regard to bacteria, but algae.
In the 1990s, scientists proposed that after corals expelled symbiotic algae during bleaching events, they might acquire new algae that were better adapted to emerging conditions. “It was a beautiful hypothesis,” Rosenberg told me. And in the years since, biologists have watched something like that occur in the Caribbean.
Coral symbionts, called zooxanthellae, primarily fall into four clades—“A” through “D.” Often, once corals recover from bleaching, scientists note that the most heat-resistant clade D tends to dominate. The change may represent an adjustment not of bacteria, but of algal symbionts better suited to higher temperatures.
Why don’t these corals harbor the heat-resistant clade D to begin with? One answer, says Tye Pettay, a research scientist at the University of Delaware, is that corals harboring this clade may grow more slowly than those with other clades. In the Orbicella corals he studies, the slower-growing, heat-resistant alga incurs a cost at normal temperatures: a greater vulnerability to erosive forces. And yet, at high temperatures, when other clades begin failing, the relative disadvantage of clade D disappears.
As sea temperatures rise, clade D seems to be advancing through the Caribbean—a probiotic wave of alga propagating through coral bodies. But clade D can’t help all corals. Many specialist corals can’t bring clade D into their tissues. That means warming conditions may leave mostly generalist corals standing in the near term, because generalists can cultivate clade D. (In the longer term, corals everywhere face ocean acidification, a potentially greater threat than rising temperatures.)
There’s an additional twist in the clade D story. Pettay recently analyzed its genome, and concluded that the alga is descended from a zooxanthellae native to a hot, shallow, often turbid region of the Indo-Pacific Ocean near Thailand.
The symbiont that’s helping Caribbean corals is, according to his analysis, an invasive species. How’d it get to the Caribbean? Pettay says that ballast water is a possible route.
If this is a probiotic wave, it’s one accidentally enabled by human hands.
“It is kind of ironic that, at least for the short term, it seems to be beneficial,” Pettay says. “I’m not going to say it’s a horrible thing, but I’m not going to say it’s a savior for reefs, either.”
We’re living through Earth’s sixth mass extinction, many argue, this one caused by human activity. Habitat loss and overharvesting aside, a major challenge is the sheer pace of change—of warming, of the human-aided spread of pathogens, of the transport of invasive species around the world. Biologists worry that plant and animal genomes can’t change quickly enough to keep up. But maybe, in some cases, their microbes can.
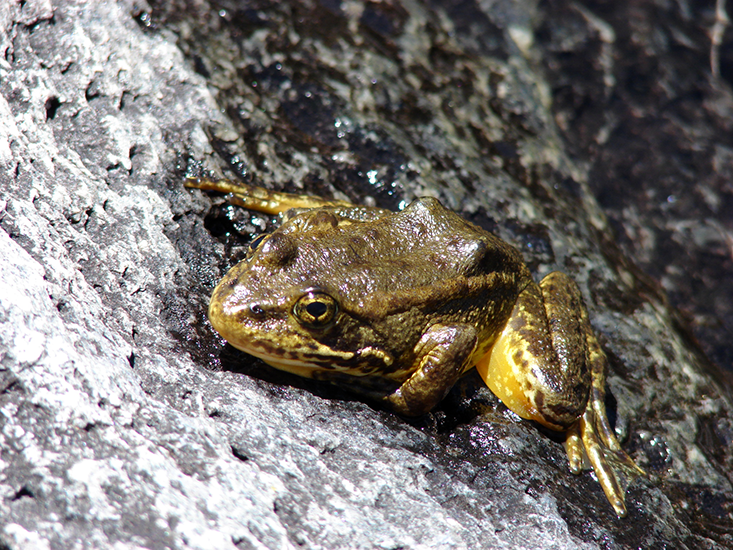
It’s unclear where the fungus that causes chytridiomycosis, an amphibian disease sometimes likened to AIDS, came from. Researchers say it may have come from southern African frogs exported in the early 20th century for use in pregnancy tests; perhaps Asian amphibians; or maybe from North American bullfrogs.
Whatever its origin, as the fungus has advanced around the globe in recent decades—and no one understands how it spreads, although perhaps it’s dispersed by birds, or even in rain drops—it has occasioned one of the worst die-offs of wildlife ever recorded. Of an estimated 7,000 amphibian species, 200 are extinct; 500 more are infected. “That’s a lot for one pathogen,” Vance Vredenburg, a professor of ecology and evolution at San Francisco State University, told me.
So it was meaningful when, in the 2000s, Vredenburg noticed that some of the amphibians he studied in California’s Sierra Nevada mountains survived the chytrid onslaught. What, he wondered, differentiated these individuals from those that died?
At a meeting in Florida, he found a clue. A biologist named Reid N. Harris gave a presentation on salamanders. He’d asked why some species nest in groups and others alone. His research had led him to an unusual explanation. Group-nesting species shared microbes that, instead of causing disease, protected their eggs from pathogenic fungi. They nested together, in other words, to share a probiotic.
Vredenburg chased Harris down after the talk. Could protective microbes explain the resistant animals he saw in the Sierra Nevada? The two scientists began to collaborate. It turned out that chytrid-resistant frogs—Vredenburg studied Rana muscosa, the mountain yellow-legged frog—tended to harbor one microbe in particular, called Janthinobacterium lividum. The microbe produced anti-fungal metabolites. It didn’t completely prevent infection, but seemed to curb fungal overgrowth. Essentially, J. lividum turned a would-be pathogen into a harmless commensal.
As a result, a strange sort of natural selection was taking place in the Sierra Nevadas. As the chytrid fungus swept through the mountains, individuals that harbored these microbes tended to survive. The chytrid pandemic was selecting frogs based on their microbes—a selective sweep in which amphibians harboring one microbial community survived above all others.
Various mammal youngsters, from elephants to horses, ingest parental feces, a practice called coprophagy.
This isn’t, of course, a “probiotic epidemic” like the one Rosenberg described in corals. One amphibian microbiome might come to dominate over time, but is wasn’t clear whether Vredenburg’s frogs were acquiring new microbes in a single generation.
The question was, could Vredenburg deliberately spark a probiotic epidemic? Could he save frogs by accelerating what was already happening naturally?
The idea was attractive because, unlike vaccines, which need to be administered to all individuals, microbes are self-propagating. Once they “stick” to one frog, they can, in theory, spread to others. An anti-chytrid microbe so acquired could be like a vaccine that’s contagious.
And for this same reason—living microbes can spread uncontrollably—Vredenburg proceeded very carefully. He and his colleagues identified a frog population that remained chytrid-free, isolated J. lividum from individuals that already carried it—precluding the possibility of introducing a new disease—and then grew the bacterium in vats.
In the interim, the fungus arrived. When Vredenburg returned to the mountains the following year, he could find just 120 frogs. He treated two-thirds, bathing each one individually in the bacterial brew, and left one-third untreated.
A year later, in 2011, when he revisited the animals, only treated individuals remained alive.
“I wouldn’t just wave the victory flag and say, ‘We got it! We got it!’ ” he told me. “We still don’t know the exact mechanism of how it works.” (And the treated frogs died the following winter, not from chytrid, but because drought reduced their over-wintering pools. They froze.) Yet Vredenburg is optimistic.
As a class, amphibians are 360 million years old, he notes. They’ve survived four mass extinctions. “This is not the first time they’ve had pathogenic fungus, I guarantee it,” he says. And microbes may have saved them before.
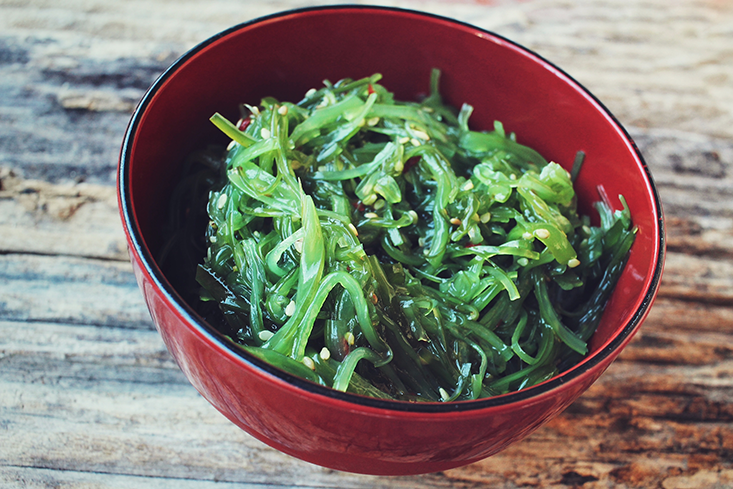
For a trait to improve fitness over evolutionary time, it must be transmissible between generations. Skeptics of the hologenome idea—that microbes and host comprise a single evolutionary unit—argue that microbes aren’t always passed like heirlooms between generations. And if they’re not, then does the holobiont really persist over evolutionary time?
Nancy Moran, an evolutionary biologist at the University of Texas, Austin, sees conceptual sloppiness in the push to generalize the hologenome idea. She agrees that microbes can improve host fitness, and even drive evolution. Her own research on pea aphids suggests as much. Aphids’ ability to tolerate warm temperatures, she has shown, depends on what strain of bacterial symbiont they harbor.
But these relationships must be proven, she says, not assumed. Microbes compete with one another, and cheating—taking more from a relationship than what you contribute—is one viable survival strategy. Some microbes are bound to adopt it. So harmony between host and microbes, or even among members of an animal’s microbiota, isn’t a given. Just because a microbe is found clinging to a host doesn’t mean it’s contributing to fitness. It might be a parasite, or merely passing through. “Of course, it’s clear that other organisms are an important part of the environment,” she says. “But it’s a big leap to go from there to the hologenome—that together they’re a unit of selection.”
Humans acquire microbes from the environment, and an openness to new microbes from the environment may help us adapt.
Proponents of the hologenome idea, meanwhile, have tried to demonstrate that microbes can drive speciation. In one experiment, Rosenberg and his colleagues at Tel-Aviv University separated fruit flies into two groups. They fed each group a different diet—molasses or sugar—and then reunited the flies after a few generations. Although still genetically similar, the flies now preferred to mate only with members of their previous group. How did they know the difference? When the scientists treated the flies with antibiotics, wiping out their microbes, they lost this preference. It was the flies’ microbiotas—not their genes—that were pushing them to diverge into separate species.
And many animals do, in fact, transfer microbes between generations. Various mammal youngsters, from elephants to horses, ingest parental feces, a practice called coprophagy. Koala mothers take it a step further: they produce a special feces called “pap” for their cubs to consume at weaning, a bequeathment of the microbes necessary to digest eucalyptus leaves.
Humans aren’t quite as obviously coprophagous, yet even we pass microbes to our progeny. Babies get a dollop from their mothers as they travel through the birth canal. And breast feeding both cultivates certain microbes, called bifidobacteria, and seeds infants with more. One recent study found that nursing mothers passed along unique, familial strains of bacteria to their infants, suggesting that, like those aphids, humans may also convey unique microbial heirlooms from mother to child.
Humans also acquire microbes from the environment, however, and the relative importance of vertical versus horizontal acquisitions isn’t entirely clear. Some evidence suggests, in fact, that an openness to new microbes from the environment may help us adapt.
Some years ago, microbiologists discovered that Japanese people harbored microbes with a unique ability to degrade seaweed, a common component of the island nation’s diet. The microbes obtained this ability, the scientists proposed, directly from seaweed-dwelling bacteria. (Bacteria can exchange bits of DNA directly.) Once procured, it’s unclear how these seaweed-degrading bacteria spread—between peers living together (horizontally), or from mother to child across generations (vertically). But the inference is that the seaweed-digesting microbes improved Japanese forebears’ fitness by enabling them to extract more nutrients from their food. The ancestors of today’s Japanese may have adapted to their unique island diet not by genetic mutation, but by microbial innovation.
Similarly, when humans first began consuming milk from domesticated animals roughly 11,000 years ago, the great majority could not digest lactose, a sugar in milk, as adults. Genes that help us digest the sugar eventually spread among milk-drinking peoples. But even today, certain populations, including some African pastoralists, regularly drink milk products without a high prevalence of “lactase persistence” genes. Microbes may aid them, some scientists hypothesize, either by pre-digesting lactose during fermentation, a common custom, or by colonizing their guts directly.
The implication is that cultural practices, like dairying, may coevolve with, and be enabled by, changes in our gut microbes. These scenarios posit the human microbiome, which we imagine as mostly confined to our gut, as actually existing in a loose network that extends beyond our bodies. It may only be by virtue of exchange with “foreign” microbial communities on, say, seaweed or in milk, that our microbiome gains new abilities, and that we thrive.
In the late 19th century, as scientists were taking in the concept of natural selection, the germ theory of disease rose to prominence. It posited that microbes, not “miasma,” caused sickness and death, and that you could cure illnesses by vanquishing the offending microbe. Seth Bordenstein, an evolutionary microbiologist at Vanderbilt University, argues that this coincidence of timing, and the power of germ theory to explain ancient human scourges such as smallpox and tuberculosis, caused biologists at that time to largely overlook how microbes might help “macrobes,” as he calls multicellular life, adapt and thrive.
Now, though, new technologies have allowed the study of microbial communities to flourish, and made it abundantly clear that macrobes are not, and never have been, alone. In this context, the hologenome idea, and the occurrence of probiotic epidemics, represent an attempt to explicitly include microbes, the dominant life form on the planet, in evolutionary theory. Understanding how microbes aid survival could have far-reaching applications, ranging from new angles on wildlife conservation to improvement of human health.
Moises Velasquez-Manoff is a journalist and author of An Epidemic of Absence: A New Way of Understanding Allergies and Autoimmune Diseases.
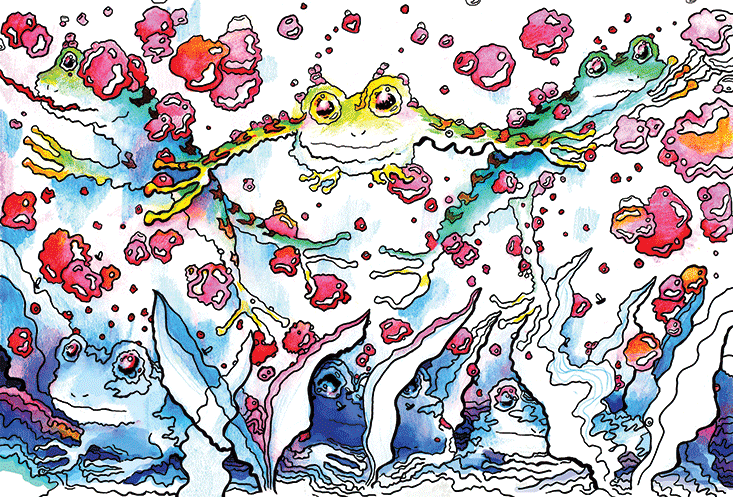