In 1926, tensions were running high at the Institute for Theoretical Physics in Copenhagen. The institute was established 10 years earlier by the Danish physicist Niels Bohr, who had shaped it into a hothouse for young collaborators to thrash out a new theory of atoms. In 1925, one of Bohr’s protégés, the brilliant and ambitious German physicist Werner Heisenberg, had produced such a theory. But now everyone was arguing with each other about what it implied for the nature of physical reality itself.
To the Copenhagen group, it appeared reality had come undone.
Bohr had electrified the scientific world in 1913 with his bold theory of how atoms are constituted. Drawing on an idea proposed in 1900 by the German physicist Max Planck, he said that the electrons that orbit the dense central nucleus are constrained to specific orbits, able to jump between them only by emitting or absorbing light in discrete packets of energy called quanta.
The theory won Bohr a Nobel Prize in 1922, but it was an ungainly, ad hoc mix of traditional physics and Planck’s new “quantum” hypothesis. Bohr craved an explanation that got to the root of why atoms seemed to behave in this peculiar way. It couldn’t be constructed from the traditional classical mechanics that had prevailed since Isaac Newton laid out its basic rules in the 17th century but demanded a new mechanics of quanta.
Such a “quantum mechanics” was devised by Heisenberg in the summer of 1925 when he was on the island of Heligoland in the North Sea, seeking relief from his dreadful hay fever. Ambitious, bold, and brilliant, Heisenberg had come to Bohr’s attention when as a student he had challenged one of Bohr’s claims in a lecture the Dane gave at Göttingen University in 1922.
Niels Bohr was pacing the room. Half a dozen physicists were shouting objections.
Bohr had invited the young man to Copenhagen in 1924, and the two of them began searching for a way to put the quantum hypothesis into a rigorous mathematical form. Heisenberg took the kind of leap of faith that Bohr had displayed in devising his quantum atom: Throw out what tradition insists on and start afresh. His scheme involved writing down the numbers measured experimentally for the frequencies of light emitted by atoms, in the form of columns called matrices. These could then be used to make calculations, for example to predict how intense the emitted rays are.
Heisenberg’s matrix-based quantum mechanics looked like the breakthrough Bohr had been waiting for. As he wrote to his own former mentor, the New Zealander Ernest Rutherford in England, “We now see the possibility of developing a quantitative theory of atomic structure.”
However, Heisenberg’s austere matrix mechanics gave no hint of what the math meant for the real world: the physical nature of matter, light, and energy. That lacuna was intentional. Heisenberg was determined to work only from what experiments had revealed, with no assumptions about the underlying reality it described.
Einstein Can’t Believe It
By 1926, the matter was a mess. The Copenhagen circle—Bohr, Heisenberg, and colleagues including the brash young Austrian Wolfgang Pauli and their collaborator Max Born, Heisenberg’s former tutor, in Göttingen—disagreed with peers such as Albert Einstein (who had proposed light quanta in 1905) and the Austrian physicist Erwin Schrödinger about how to interpret the new quantum mechanics. The suggestion coming from Copenhagen that the quantum world simply couldn’t be visualized in terms of objects interacting in time and space seemed to Einstein and Schrödinger to relinquish all that science strove to attain: a physical picture of reality.
What’s more, in the early part of the year, Schrödinger announced a rival form of quantum mechanics to Heisenberg’s, based on the idea that quantum particles could be described as waves. That seemed to restore a physical picture of what was going on: Matrices were abstract and mathematical, but waves—even if they were waves of particle-like entities like electrons—were familiar aspects of reality. But Heisenberg, dismayed by this apparent competition for a true quantum theory, dismissed wave mechanics as “crap”; Schrödinger in turn said he was “repelled” by the abrupt quantum jumps between energy states assumed in matrix mechanics.
Soon, however, the Copenhagenists didn’t even agree with each other. In trying to make sense of Heisenberg’s ideas, Bohr found himself at loggerheads with the young German. Bohr was inclined to accept Schrödinger’s waves and to make a concept called “complementarity,” the keystone of quantum mechanics: Entities like electrons could behave like particles or waves, but not at the same time or in the same experiment.
Heisenberg didn’t care for such qualitative ideas, wanting instead just to stick with the math. “Our talks often continued till long after midnight and did not produce a satisfactory conclusion despite protracted efforts,” he wrote later. “Both of us became utterly exhausted and rather tense.” Their disagreements almost reduced Heisenberg to tears.
In the spring of 1927, Bohr took off on a skiing trip in Norway to think the matter over by himself. In his absence, Heisenberg wrote a paper in which he attempted to explain what his matrix mechanics implied for quantum objects. What he concluded seemed bizarre.
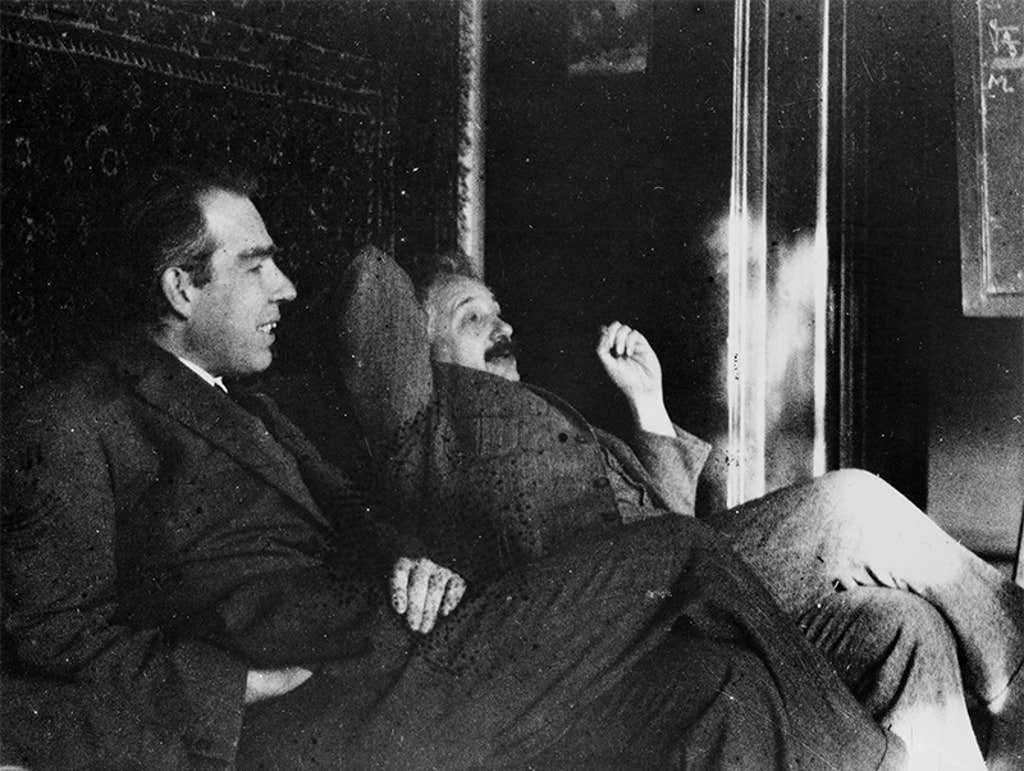
There are some pairs of properties of quantum particles, he said, that we can never measure simultaneously as accurately as we like. If we measure one of them more accurately, the other necessarily becomes less certain. There is, Heisenberg said, a fundamental uncertainty at the heart of nature. From the perspective of classical physics this seems crazy, for there is nothing to stop us measuring at once the speed and position of a large classical object (how could speeding fines ever be imposed if there were?).
Heisenberg submitted his paper for publication while Bohr was on the ski slopes, only to find on his boss’ return that Bohr thought it contained serious flaws. Heisenberg sought to illustrate his “uncertainty principle” by showing how an attempt to measure the position and speed of an electron by bouncing a gamma ray off it would inevitably disturb the very things one was trying to measure. But Bohr felt that here Heisenberg was overlooking the wavy character of the gamma ray—as he was, of course, because he didn’t like the idea of waves in quantum mechanics at all.
More heated conversations followed, after which Heisenberg agreed to add a note to the end of the paper acknowledging Bohr’s view on the complementarity of waves and particles. “Thank God!” he wrote to his parents. “My friendship with Bohr is of course more important than physics.”
What was at stake was more than whether the new quantum mechanics was right or not. To the Copenhagen circle—here, at least, Bohr and Heisenberg agreed—the theory challenged the assumptions underlying the entire scientific enterprise for the past several centuries.
Quantum mechanics, they said, demanded we throw away the old reality and replace it with something fuzzier, indistinct, and disturbingly subjective. No longer could scientists suppose that they were objectively probing a pre-existing world. Instead, it seemed that the experimenter’s choices determined what was seen—what, in fact, could be considered real at all.
In other words, the world is not simply sitting there, waiting for us to discover all the facts about it. Heisenberg’s uncertainty principle implied that those facts are determined only once we measure them. If we choose to measure an electron’s speed (more strictly, its momentum) precisely, then this becomes a fact about the world—but at the expense of accepting that there are simply no facts about its position. Or vice versa.
But the question, “What were the position or momentum before we measured one or the other?” has no answer—not because we couldn’t know until we looked, but because the question has no meaning. We seem to choose what is real. This idea seemed crazy to Einstein. Heisenberg said Einstein refused to admit that “it was impossible, even in principle, to discover all the partial facts needed for the complete description of a physical process. And so he refused point-blank to accept the uncertainty principle.”
Einstein was exasperated. How could the moon only be real if he looked at it?
A century later, scientists are still arguing about this issue of what quantum mechanics means for the nature of reality. Alternative interpretations of quantum mechanics have arisen and generated their own heated debates.
One of these is the “pilot-wave” theory, a form of which was presented by the French physicist Louis de Broglie in the 1920s but which was more fully developed in the 1950s by David Bohm, and which says that quantum particles are real entities with well-defined properties in time and space, but which move and interact under the influence of waves in a somewhat mysterious field called the quantum potential.
Perhaps the most popular alternative interpretation is typically dubbed the many-worlds view, first proposed by the American physicist Hugh Everett in 1957, which argues that measurements don’t impose a choice on which of the possible outcomes allowed by quantum mechanics become “real”; rather, all outcomes are realized, but in different worlds that diverge when the measurement is made.
Physicist Anton Zeilinger, who shared the 2022 Nobel Prize for his experimental studies of the elusive nature of the quantum world, said it’s impossible to demonstrate “that any particle has a realistic trajectory,” in the case of the Broglie-Bohm interpretation. Meanwhile, Everett’s claim “that the observer coexists in many different states is intrinsically untestable.”
Such alternative interpretations of quantum behavior suggest that physicists want objective reality back. They want a return to what philosophers call realism, where things have definite positions and properties irrespective of how or whether we look at them. But so far, scientific investigations of the quantum world have not obliged the demands of the realists.
Bohr Won’t Let Schrödinger Sleep
At first, Heisenberg’s matrix mechanics was too much even for some of his Copenhagen colleagues. The math was fine, but what jarred was Heisenberg’s insistence that it was meaningless to seek a physical picture of what was going on. Forget the whole idea of electron orbits in atoms, he said—that’s just a manner of speaking. But, Pauli objected, we can see the moon following an orbit! How can electrons be any different?
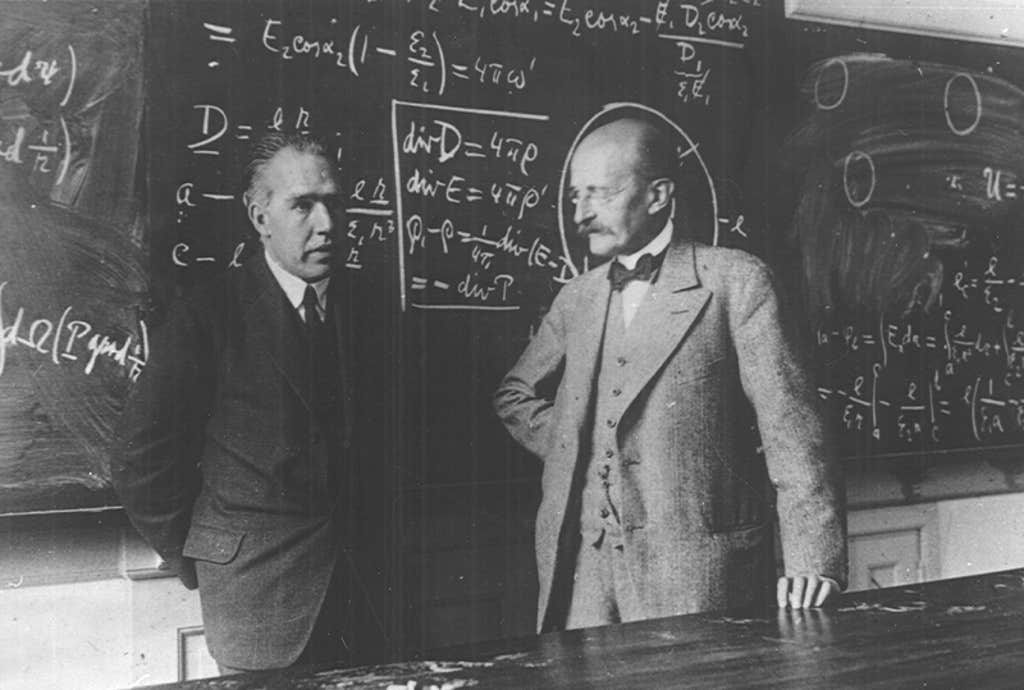
Bohr, on the other hand, seemed to delight in the puzzle. “How wonderful that we have met with a paradox,” he said. “Now we have some hope of making progress.” That was Bohr all over: to embrace a contradiction as if it was an answer. It was almost as if, by merely coining a word for it—complementarity—Bohr was claiming to have solved the problem. Complementarity might be loosely said to imply that contradictory things can both be true, but just not at the same time. A particle can be a wave, and vice versa—but not both at once. “The opposite of one profound truth may very well be another profound truth,” Bohr was fond of saying.
Bohr has been justifiably criticized for expressing his ideas obscurely, both in writing and in his lectures. But that seems to be because he was struggling to express ideas that our everyday language isn’t designed to capture. When we say that wavelike quantum particles can be “in many places or states at once,” or that a measurement of some property of a particle “collapses” all its smeared-out waviness into a single value, or that the strange quantum phenomenon called entanglement makes a measurement on one particle instantly influence another no matter how far away it is, we’re not really talking about what quantum mechanics is like but are constructing pictures of it that are based on our own classical intuitions—exactly what quantum physics undermines.
Quantum mechanics replaced the old reality with something disturbingly subjective.
Schrödinger felt that his wave-based quantum mechanics offered an escape from the indistinct, unformed reality that seemed to emerge from Copenhagen. Sure, it was weird that quantum particles could behave like waves, as though they were smeared out in space—but once you got over that, at least the waves felt like something real. But when Schrödinger visited Copenhagen in the autumn of 1926 to discuss his theory, he did not quite reckon with the reception he would get. Bohr later said, rather blandly, that “Schrödinger gave us a most impressive account of his wonderful work.” In fact, it was a raging battle.
Schrödinger held a seminar with the Copenhagen team that apparently descended into turmoil. According to one account, “Half a dozen physicists were shouting objections and questions. Bohr, his pipe forgotten, was pacing the room. Everyone was haranguing his neighbor … the commotion went on for most of the week.”
Apparently exhausted by the arguments, Schrödinger caught a cold and went to bed with a fever at the Institute’s guest rooms. That brought no respite. Bohr continued to debate the matter at Schrödinger’s bedside until Bohr’s wife Margrethe persuaded him to relent for the sake of their guest’s fragile health.
“If I had known that [the wave theory] was going to be taken so seriously as to cause all of this discussion,” Schrödinger said at one point, “I would never have invented it.” And if in contrast we were forced to accept the abominable quantum jumps alleged by Bohr, Heisenberg, and their colleagues, “I should be sorry I ever got involved with quantum theory.”
But Bohr responded by assuring him that everyone else was grateful that he had—because Schrödinger’s wave mechanics “represents a gigantic advance over all previous forms of quantum mechanics.” He wasn’t just trying to placate his guest; to Heisenberg’s chagrin, Bohr and many of his colleagues adopted Schrödinger’s wave calculus in preference to Heisenberg’s matrices, as it was generally easier to use. Schrödinger himself proved that both versions of quantum mechanics are equivalent, so which one uses is just a matter of taste.
At any rate, Schrödinger’s wave mechanics didn’t restore the kind of reality he and Einstein wanted. His theory represented all that could be said about a quantum object in the form of a mathematical expression called the wave function, from which one can predict the outcomes of making measurements on the object. The wave function looks much like a regular wave, like sound waves in air or water waves on the sea. But a wave of what?
At first, Schrödinger supposed that the amplitude of the wave—think of it like the height of a water wave—at a given point in space was a measure of the density of the smeared-out quantum particle there. But Born argued that in fact this amplitude (more precisely, the square of the amplitude) is a measure of the probability that we will find the particle there, if we make a measurement of its position.
Heisenberg’s Most Disconcerting Implication
This so-called Born rule goes to the heart of what makes quantum mechanics so odd. Classical Newtonian mechanics allows us to calculate the trajectory of an object like a baseball or the moon, so that we can say where it will be at some given time. But Schrödinger’s quantum mechanics doesn’t give us anything equivalent to a trajectory for a quantum particle. Rather, it tells us the chance of getting a particular measurement outcome. It seems to point in the opposite direction of other scientific theories: not toward the entity it describes, but toward our observation of it. What if we don’t make a measurement of the particle at all? Does the wave function still tell us the probability of its being at a given point at a given time? No, it says nothing about that—or more properly, it permits us to say nothing about it. It speaks only to the probabilities of measurement outcomes.
Crucially, this means that what we see depends on what and how we measure. There are situations for which quantum mechanics predicts that we will see one outcome if we measure one way, and a different outcome if we measure the same system in a different way. And this is not, as is sometimes implied (this was the cause of Heisenberg’s row with Bohr), because making a measurement disturbs the object in some physical manner, much as we might very slightly disturb the temperature of a solution in a test-tube by sticking a thermometer into it. Rather, it seems to be a fundamental property of nature that the very fact of acquiring information about it induces a change.
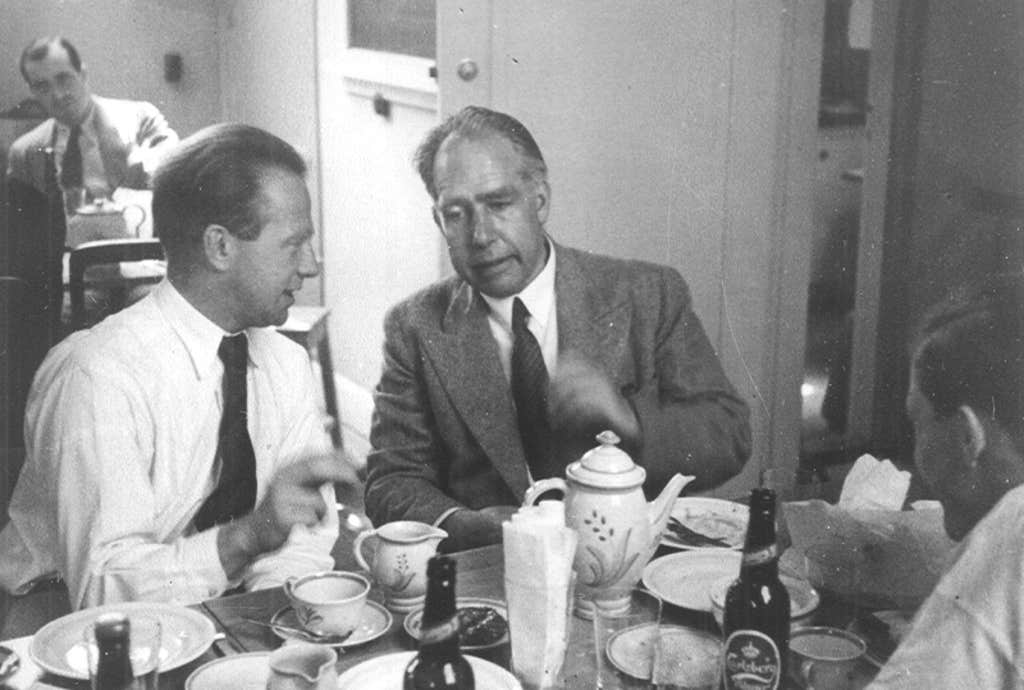
If, then, by reality we mean what we can observe of the world (for how can we meaningfully call something real if it can’t be seen, detected, or even inferred in any way?), it is hard to avoid the conclusion that we play an active role in determining what is real—a situation the American physicist John Archibald Wheeler called the “participatory universe.” This view seemed absurd to Einstein, who asked in exasperation how the moon could only be real if he looked at it.
Einstein was right to be disturbed by all this. How could you not be, if you truly appreciated what the message from Copenhagen was? When, after one discussion, someone told Bohr that it made them giddy to think about the implications, he responded, “But if anybody says he can think about quantum problems without getting giddy, that only shows that he has not understood the first thing about them.”
Heisenberg’s “uncertainty” captured that sense of the ground shifting. It was not the ideal word—Heisenberg himself originally used the German Ungenauigkeit, meaning something closer to “inexactness,” as well as Unbestimmtheit, which might be translated as “undeterminedness.” It was not that one was uncertain about the situation of a quantum object, but that there was nothing to be certain about.
There was an even more disconcerting implication behind the uncertainty principle. The vagueness of quantum phenomena, when an electron in an atom might seem to jump from one energy state to another at a time of its own choosing, seemed to indicate the demise of causality itself. Things happened in the quantum world, but one could not necessarily adduce a reason why. In his 1927 paper on the uncertainty principle, Heisenberg challenged the idea that causes in nature lead to predictable effects. That seemed to undermine the very foundation of science, and it made the world seem like a lawless, somewhat arbitrary place.
Alternative interpretations suggested physicists wanted objective reality back.
Reality, previously a world in which well-defined particles interacted through precise laws, so that in principle the future could be predicted from a complete knowledge of the present, seems to dissolve into a haze of possibilities, within which there was no longer any objective viewpoint for an observer to stand. The position that denies any pre-existing reality, independent of our knowledge of it, is called anti-realism. Was this then really what the Copenhagen interpretation amounted to?
Some think so, but it’s not obvious. Regarding quantum mechanics as a theory about the probabilities of measurement outcomes is not the same as saying that there is nothing before a measurement is made. It is simply to recognize the limitations of what the theory permits us to say about that.
One might say that, in this view, the theory doesn’t speak about how things are, but about what we will see if we observe. It doesn’t deny the independent existence of some substrate of the world that gives rise to the observations, but only of our right to pronounce on it. Historian of science Mara Beller puts it another way: Bohr’s position implies that we do not need to adopt a realist view of the world to use quantum mechanics to make predictions about what we will observe.
Properly construed (and the Copenhagen team were not always themselves sufficiently careful to make this clear) the interpretation shows us the limits of what, if quantum mechanics is correct, we can conclude with confidence about reality. That’s the austerity that Zeilinger advocates in any search for deeper understanding.
The problem was that Bohr, Heisenberg, and their collaborators tended to imply that such a search was pointless; we just had to accept that this is how things are. It’s no wonder Einstein and others tried so hard to restore some version of the old objective reality.
One of Bohr’s most provocative views was that there is a fundamental distinction between the fuzzy, probabilistic quantum world and the classical world of real objects in real places, where measurements of, say, an electron with a macroscopic instrument tell us that it is here and not there.
What Bohr meant is shocking. Reality, he implied, doesn’t consist of objects located in time and space. It consists of “quantum events,” which are obliged to be self-consistent (in the sense that quantum mechanics can describe them accurately) but not classically consistent with one another. One implication of this, as far as we can currently tell, is that two observers can see different and conflicting outcomes from an event—yet both can be right.
But this rigid distinction between the quantum and classical worlds can’t be sustained today. Scientists can now conduct experiments that probe size scales in between those where quantum and classical rules are thought to apply—neither microscopic (the atomic scale) nor macroscopic (the human scale), but mesoscopic (an intermediate size). We can look, for example, at the behavior of nanoparticles that can be seen and manipulated yet are small enough to be governed by quantum rules. Such experiments confirm the view that there is no abrupt boundary of quantum and classical. Quantum effects can still be observed at these intermediate scales if our devices are sensitive enough, but those effects can be harder to discern as the number of particles in the system increases.
To understand such experiments, it’s not necessary to adopt any particular interpretation of quantum mechanics, but merely to apply the standard theory—encompassed within Schrödinger’s wave mechanics, say—more expansively than Bohr and colleagues did, using it to explore what happens to a quantum object as it interacts with its surrounding environment. In this way, physicists are starting to understand how information gets out of a quantum system and into its environment, and how, as it does so, the fuzziness of quantum probabilities morphs into the sharpness of classical measurement. Thanks to such work, it is beginning to seem that our familiar world is just what quantum mechanics looks like when you are 6 feet tall.
But even if we manage to complete that project of uniting the quantum with the classical, we might end up none the wiser about what manner of stuff—what kind of reality—it all arises from. Perhaps one day another deeper theory will tell us. Or maybe the Copenhagen group was right a hundred years ago that we just have to accept a contingent, provisional reality: a world only half-formed until we decide how it will be.
Lead image of Werner Heisenberg, Erwin Schrödinger, and Niels Bohr by Tasnuva Elahi with images by Natata, MM memo, Night_Lynx, and Naci Yavuz / Shutterstock