The novelist William Golding suggested to James Lovelock that he name his now-famous hypothesis after the Greek goddess of the Earth, Gaia. It was a good fit: Lovelock believed that the living and inanimate parts of the Earth formed a single, interacting, and self-regulating system. Lovelock’s work grew, in part, out of research he had done for NASA, and published in a 1965 Nature paper, about the signs of life we might look for on other planets.
Forty-six years later, NASA’s Kepler Space Telescope discovered the planet Kepler-22b. It was one of the first planets outside of our solar system confirmed orbiting a sun-like star at a distance that would allow for liquid water to exist on its surface. The availability of liquid water, which is essential to every form of life that we know of, would raise the chances that the planet harbors life. Also, Kepler-22b’s estimated density suggests that it may be composed of rock, which means it might be able to reproduce conditions similar to those found on Earth.
Now, scientists are beginning to understand the importance of another characteristic of any planet likely to support life, and it is one that fits squarely into Lovelock’s view of Earth as a dynamic participant rather than a passive backdrop: an active system of drifting continents, otherwise known as plate tectonics. On the face of it, the connection between life, with its relatively brief cycles and dizzying complexity, and plate tectonics, with its much slower cycles and ostensibly simpler interactions, appears tenuous. But on Earth, at least, that connection is direct and deep.
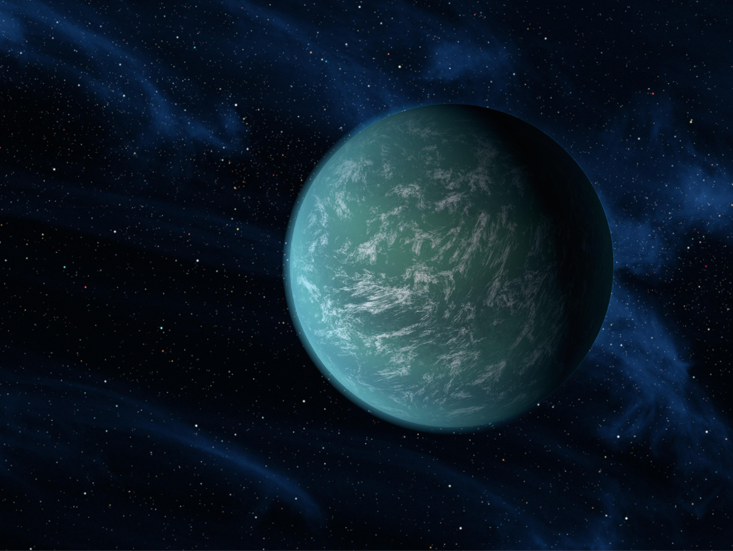
Earth’s continents move because they sit on separate plates, composed of the crust and upper mantle, which float like islands on a “sea” of hot, fluid-like rock below. The motions of these plates are part of a giant machine that constantly shuttles water, carbon dioxide, and other ingredients vital for life from the deep interior to the terrestrial surface and atmosphere, and back again. Thanks to plate tectonics, “the elements on the periodic table are continually being resupplied to the land, lakes, and oceans,” notes Stanford geophysicist Norman Sleep. Life depends on these cycles in countless ways.
Furthermore, without plate tectonics, our planet wouldn’t have a climate stable enough to support life over the billions of years it’s been evolving. That’s because plate tectonics is an essential part of a crude planetary thermostat called the “carbonate-silicate” cycle.1, 2 Carbon dioxide in the atmosphere dissolves in rainwater to form carbonic acid, which dissolves silicate rocks. The byproducts of this erosion, or “weathering,” are carried to the oceans where they are ingested by organisms—such as tiny planktonic foraminifera—and incorporated into limestone (calcium carbonate) shells. When those creatures die, they fall to the bottom of the ocean and pile up as sediments.
At plate boundaries, some of these sediments are carried down even further through subduction (when one plate slides under another). Under the conditions of higher temperature and pressure found in the deep earth, carbon dioxide gas is released, eventually making its way to the atmosphere through volcanoes, thereby completing the cycle.
When the Earth’s temperature increases, more water evaporates from the surface, leading, in turn, to more precipitation, which washes more carbon dioxide out of the atmosphere and sends it deep underground through tectonic pathways. This diminishes the greenhouse effect, leading to global cooling. Conversely, when the Earth cools, less carbon dioxide rains out and atmospheric concentrations build up from volcanoes and other sources, leading to a strengthened greenhouse effect and eventual warming. Tilman Spohn, who directs the Institute of Planetary Research at the German Aerospace Center in Berlin, calls plate tectonics “the most efficient known mechanism for recycling” of this sort.
Removing life from the equation led to a very
different-looking planet.
Remarkably, the dependence of life on plate tectonics is reciprocal: Scientists have come to realize that plate tectonics relies on life just as much as life relies on it. “Life may stabilize plate tectonics, which, in turn, may stabilize the habitability of a planet,” says Spohn.
An important part of the feedback from biology to geology stems from the “deep water” cycle, which is closely tied to weathering. Life on the Earth’s surface contributes to weathering in a variety of ways, from bacterial acid secretion that breaks down rocks, to the growth on rocks of lichens, moss, and trees. “The sedimentation rate is a function of the activity of the biosphere,” Spohn claims. In other words, the more life there is on a planet, the more weathering there will be.
The sediments that result from this process accumulate on the ocean floor, and contain up to 40 percent water by weight.3 When subduction occurs at plate boundaries, they are conveyed down into the mantle, about 62 miles (100 kilometers) beneath the Earth’s surface.
The water tied up in these subsurface strata in turn serves as a kind of lubricant that makes plate motions possible. That’s because water can break the bonds that hold rocks together, making them more malleable and fluid-like. “If a planet doesn’t have water in its mantle,” Spohn explains, “it wouldn’t have plate tectonics, which involves the slow movement of mantle rock up towards the surface. Instead you’d have a stagnant layer of rocks on top.” That’s what we see on Mercury and the moon.
Increasing the water content of rock also lowers its melting point. Delivering more water to the mantle will therefore generate more molten material, leading to more volcanic eruptions. “The outflow of this volcanic rock is basically the stuff that makes continental crust,” which mainly consists of granite, says Spohn. “The more of this melt that you produce, the more the continents are growing.” Given that a thriving biosphere can result in more water reaching the mantle, he surmises, it can also contribute to the growth of continents.4
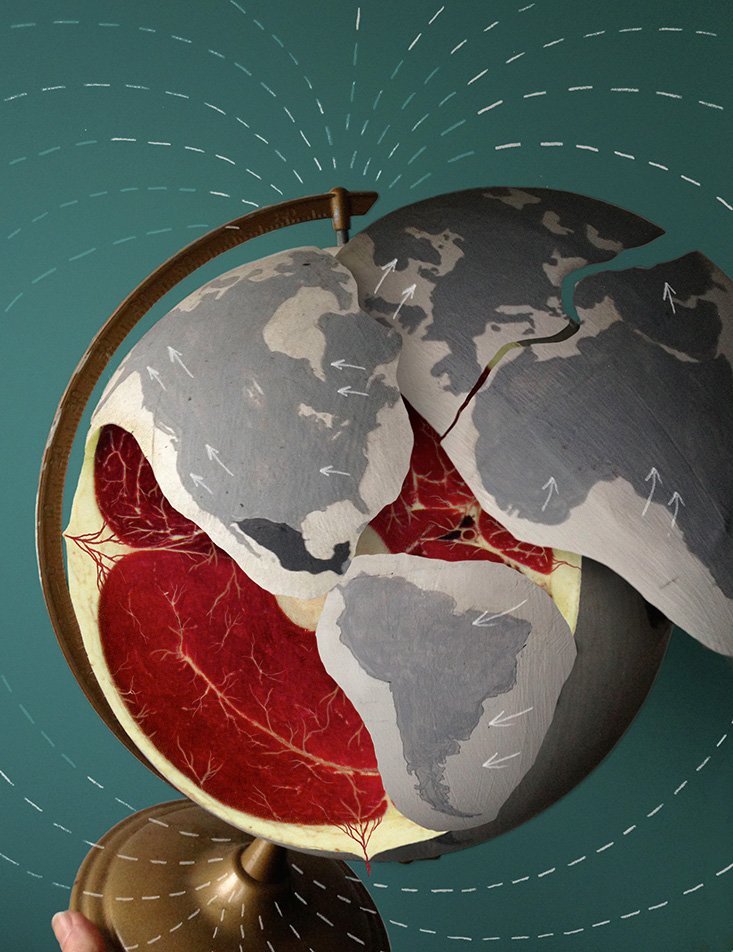
In 2013, Spohn, Dennis Höning, and two other colleagues imagined what Earth might look like without plate tectonics. They used computer simulations to spin out two very different scenarios—one with an active biosphere in the mix and one without. In the “biotic” case, biological weathering contributed to enhanced sedimentation, resulting in about 300 parts per million of water in the mantle. This, in turn, led to more frequent volcanic outbursts and continents covering about 40 percent of the Earth’ surface, roughly comparable to the world we see today.
Removing life from the equation led to a very different-looking planet. Reduced sedimentation resulted in lower concentrations of water in the mantle (only about 40 parts per million) and a correspondingly diminished production of continental crust. A lifeless Earth, the model predicts, would essentially be a water world with little landmass—constituting perhaps as little as 3 percent of the surface.
“We can’t say which came first, plate tectonics or life,” Spohn says. “What we can say is that they basically stabilize each other. If we suddenly switched off plate tectonics, it wouldn’t be very good for us. And if we switched off life, it wouldn’t be good for plate tectonics.”
The idea that plate tectonics makes life more likely is beginning to steer the search for extraterrestrial life. Northwestern University astronomer Nicolas Cowan and his colleagues recently demonstrated a technique for detecting the presence of oceans and continents on an exoplanet. So far they’ve only tested out the approach on Earth, using a telescope on NASA’s Deep Impact spacecraft that was tens of millions of miles away when it made its observations.5 The basic idea is to see how the color of a planet changes over a time as it rotates on its axis.
After looking at the Earth for 24 hours with Deep Impact’s small telescope, Cowan’s team was able to make a map of the planet that had three colors: blue corresponding to the oceans, orange corresponding to the land, and gray corresponding to clouds.6 Longer-term observations would show the gray patches (or clouds) moving around, so that the scientists could get a more refined picture of where the oceans and continents lie.
This technique has not yet been applied to remote planets, but Cowan believes it might soon help astrobiologists decide which exoplanets to focus on. “If a planet has large continents and large bodies of water, it’s hard to make that without plate tectonics,” he says. And plate tectonics are less likely to exist without life.
Steve Nadis, a writer based in Cambridge, Mass., is a contributing editor to Astronomy and Discover magazines. He is coauthor of A History in Sum: 150 Years of Mathematics at Harvard.
References
1. Kasting, J.F. & Catling, D. Evolution of a habitable planet. Annual Review of Astronomy and Astrophysics 41, 429-463 (2003).
2. Walker, J.C.G., Hays, P.B., & Kasting, J.F. A negative feedback mechanism for the long-term stabilization of Earth’s surface temperature. Journal of Geophysical Research 86, 9776-9782 (1981).
3. Honing, D., Hansen-Goos, H., Airo, A., & Spohn, T. Biotic vs. abiotic Earth: A model for mantle hydration and continental coverage. Planetary and Space Science (2013). Retrieved from http://dx.doi.org/10.1016/j.pss.2013.10.004.
4. Rosing, M.T., Bird, D.K., Sleep, N.H., Glassley, W., & Albarede, F. The rise of continents—An essay on the geologic consequences of photosynthesis. Palaeogeography, Palaeoclimatology, Palaeoecology 232, 99-113 (2006).
5. Steigerwald, B. EPOXI Team Develops New Method to Find Alien Oceans. NASA.gov (2009).
6. Cowen, N.B., et al. Alien maps of an ocean-bearing world. The Astrophysical Journal 700, 915-923 (2009).