On a late summer evening in 1961, biochemist Osamu Shimomura was nearing the end of another frustrating day working with the jellyfish Aequorea victoria at the University of Washington’s Friday Harbor Laboratories.
For weeks he’d been trying to pin down the enzyme that causes A. victoria, also known as the crystal jelly, to give off a bioluminescent glow when disturbed. This enzyme, one of a group called luciferases (lucifer means “light bearer”), produces a highly energized molecule that quickly releases its energy as light—but as of yet, nobody had been able to isolate it from the jellyfish.
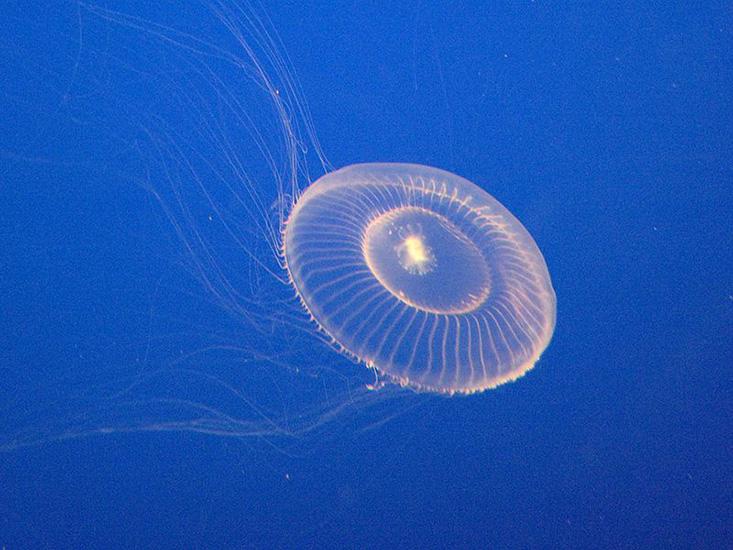
Shimomura, who had years of experience working with bioluminescent organisms, spent the afternoon testing whether increasing or decreasing the acidity of his samples would highlight the presence of the luciferase. Some of his tweaks produced a weak glow—but none offered the fireworks he was looking for. Calling it a day, he tossed the remnants of his work into a nearby sink. Suddenly there was what he would later describe as a “bright blue flash.” Something in that sink had set the biological lanterns in the samples alight far more effectively than anything else Shimomura had done.
It didn’t take long to identify the catalyst: The sink where he’d discarded his crystal jelly samples contained seawater draining from nearby tanks, and calcium in the seawater had set the discarded material aglow. With a nod toward the scientific name of his research animal, Shimomura called the protein aequorin.
But if aequorin produced the blue light that Shimomura saw that afternoon, why did the crystal jelly glow green when it was disturbed? Perhaps, Shimomura speculated, another protein was absorbing energy from the blue light and using it to produce green light. Some further experiments proved his hypothesis was correct. Shimomura had discovered green fluorescent protein, or GFP. The possible implications of this discovery were enormous, but the world had to wait for technology to catch up and turn those possibilities into realities.
In the mid-1980s, a Columbia University biochemist named Martin Chalfie was using the lab worm Caenorhabditis elegans in an attempt to isolate genes associated with specific nerve cells and determine where the worm used them. It was an arduous, time-consuming process that involved immobilizing, or fixing, the animals at various stages of life and then probing the specimens for indirect evidence of gene expression.
Then, one afternoon, he happened to attend a lecture by an expert in bioluminescence named Paul Brehm, who mentioned Shimomura’s discovery of a light-activated glowing green protein in a marine jelly. Chalfie immediately recognized how GFP could help him. Since C. elegans, like the crystal jelly, is essentially transparent, inserting GFP into his worms would allow Chalfie to use the glowing protein as markers to show when specific proteins were being used. All Chalfie needed was the genetic sequence for GFP.
Something in that sink had set the biological lanterns in the samples alight.
He learned that Douglas Prasher, a researcher at Woods Hole Oceanographic Institute at the time, was working on cloning and sequencing GFP—but before they could collaborate, Chalfie went on sabbatical and the researchers lost touch. A few years later, Ghia Euskirchen, a chemical-engineering graduate student with expertise in fluorescence, joined Chalfie’s team, and soon turned up a recent paper on GFP by Prasher. After Chalfie and Prasher reconnected, Prasher sent along the precious DNA.
From there, Chalfie’s team took the first big step toward using GFP as a protein tracer in living cells. First, they got E. coli to make GFP and glow green under ultraviolet light, proving that the molecule could be a stand-alone marker. At the same time and on the other side of the continent, a University of California, San Diego, biochemist named Roger Tsien was tweaking GFP to create versions that fluoresce different colors and to improve on GFP’s brightness and stability, making it even better at illuminating the cellular and organismal location of a protein that is partnered with it.
Then, in 1994, Columbia professor Tulle Hazelrigg, who is married to Chalfie, successfully linked the genes for GFP to another protein while preserving the function of both. This fusion construct, which Hazelrigg demonstrated in fruit flies, has become one of the most critical applications of GFP in science today. Finally, as Chalfie had envisioned, the green glow of GFP could accompany the target protein anywhere the organism used it.
This work on GFP proved so essential that Chalfie, Shimomura, and Tsien won the Nobel Prize in Chemistry in 2008. By then, a lack of funding had led Prasher, who’d been the first person to clone GFP, to quit scientific research altogether. (He was driving a courtesy shuttle for a car dealership in Alabama at the time.) Later he joined the lab of Tsien, who considered Prasher’s contributions to the GFP story “irreplaceable.” Tsien passed away in 2016.
Today, Shankar Chinta, of Touro University California, and Manish Chamoli, at the Buck Institute for Research on Aging, are using GFP to track disease-associated proteins in C. elegans and other organisms. They’re on the lookout for when proteins start to clump together; this type of aggregating in the brain is a hallmark of Parkinson’s, Huntington’s, and other aging-related diseases. “We have selected five or six genes,” Chamoli says, “the ones expressed throughout the body, so we can see how the [protein] aggregation changes.” According to Chinta, tracing the whens and whys of protein aggregation will highlight targets for potential therapies. “Once we understand the basic biology of these aggregates,” he says, “we can screen for compounds that can actually prevent aggregation.”
The applications of GFP aren’t limited to tracing diseases associated with aging. This protein also helps scientists track cancer cells, trace complex neuronal circuits using a rainbow of fluorescent colors, and follow viruses as they infect cells. But the original question that got us to GFP wasn’t, “What can it do for us?” Instead, our human curiosity led us to ask, simply, “Why does it glow?” Now, the molecule that answered that basic question helps us illuminate the secrets of human health.
Emily Willingham is a journalist, Forbes contributor, and biology professor. She is co-author with Tara Haelle of The Informed Parent: A Science-Based Resource for Your Child’s First Four Years.
This story was commissioned by the Science Philanthropy Alliance as part of its Science to Society series, illustrating the importance of basic scientific research.