The universe has cooked up all sorts of bizarre and beautiful forms of matter, from blazing stars to purring cats, out of just three basic ingredients. Electrons and two types of quarks, dubbed “up” and “down,” mix in various ways to produce every atom in existence.
But puzzlingly, this family of matter particles—the up quark, down quark and electron—is not the only one. Physicists have discovered that they make up the first of three successive “generations” of particles, each heavier than the last. The second- and third-generation particles transform into their lighter counterparts too quickly to form exotic cats, but they otherwise behave identically. It’s as if the laws of nature were composed in triplicate. “We don’t know why,” said Heather Logan, a particle physicist at Carleton University.
In the 1970s, when physicists first worked out the Standard Model of particle physics—the still-reigning set of equations describing the known elementary particles and their interactions—they sought some deep principle that would explain why three generations of each type of matter particle exist. No one cracked the code, and the question was largely set aside. Now, though, the Nobel Prize-winning physicist Steven Weinberg, one of the architects of the Standard Model, has revived the old puzzle. Weinberg, who is 86 and a professor at the University of Texas, Austin, argued in a January 2020 paper in the journal Physical Review D that an intriguing pattern in the particles’ masses could lead the way forward.
“Weinberg’s paper is a bit of lightning in the dark,” said Anthony Zee, a theoretical physicist at the University of California, Santa Barbara. “All of a sudden a titan in the field is suddenly working again on these problems.”
“I’m very happy to see that he thinks it’s important to revisit this problem,” said Mu-Chun Chen, a physicist at the University of California, Irvine. Many theorists are ready to give up, she said, but “we should still be optimistic.”
The Standard Model does not predict why each particle has the mass that it does. Physicists measure these values experimentally and manually plug the results into the equations. Measurements show that the minuscule electron weighs 0.5 megaelectron volts (MeV), while its second- and third-generation counterparts, called the muon and the tau particle, tip the scales at 105 and 1,776 MeV, respectively. Similarly, the first-generation up and down quarks are relative lightweights, while the “charm” and “strange” quarks comprising the second quark generation are middleweights, and the “top” and “bottom” quarks of the third generation are heavy, the top weighing a monstrous 173,210 MeV.
The spread in the masses is vast. When physicists squint, though, they see a tantalizing structure in where the masses fall. The particles cluster into somewhat evenly spaced generations: The third-generation particles all weigh thousands of MeV, second-generation particles weigh roughly hundreds of MeV, and first-generation particles come in at around an MeV each. “As you go each level down, they get exponentially lighter,” says Patrick Fox, a particle physicist at the Fermi National Accelerator Laboratory in Illinois.
In the equations of the Standard Model, the mass of each particle corresponds to the degree to which it “feels” a universe-filling field known as the Higgs field. Top quarks are heavy because they experience intense drag as they move through the Higgs field, like a fly stuck in honey, while wispy electrons flit through it like butterflies in air. In this framework, how each particle feels the field is an intrinsic attribute of the particle.
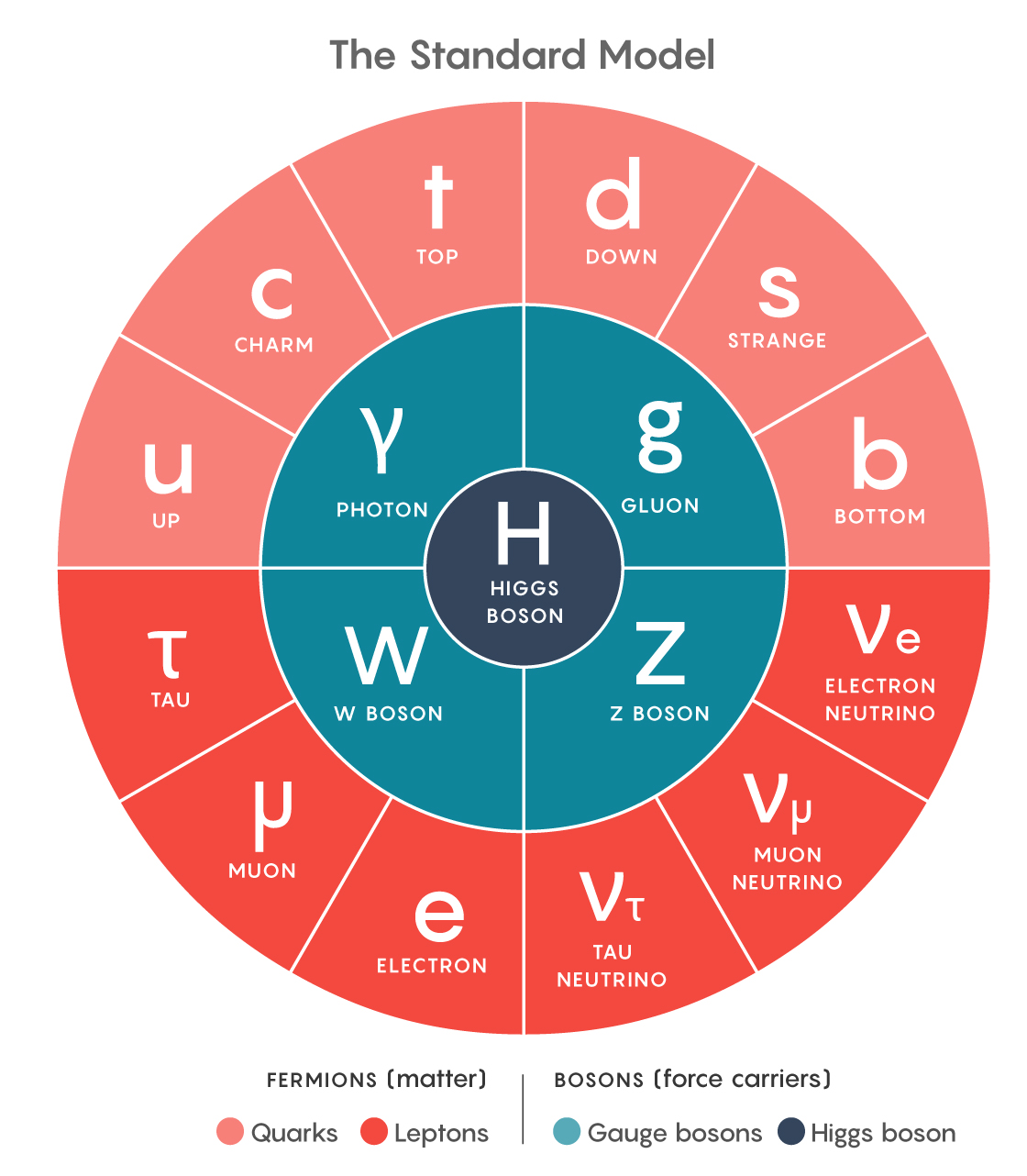
In the heady days of the Standard Model’s youth, explaining where these attributes came from was seen as the next logical step. Zee recalls asking his then-graduate student Stephen Barr to calculate the mass of the electron as his doctoral project—a task Weinberg’s January paper struggles with today, more than 40 years later. Barr and Zee published a rough idea in 1978, but string theory exploded onto the scene just a few years later, Zee says, sweeping away such efforts.
Barr and Zee’s main idea, partly inspired by Weinberg’s earlier works, was to follow the mass. Compared with the top quark’s ponderous bulk, the masses of the electron and other particles look like rounding errors. Perhaps that’s because they are. Barr and Zee suggested that only the heft of the heavier particles is fundamental in some sense.
A 2008 theory by Fox and Bogdan Dobrescu of Fermilab picked up where they left off. The top quark’s mass happens to be roughly the same as the average energy of the Higgs field, so Fox and Dobrescu assumed that only the top quark slogs through the field in the standard way. “The top is clearly special in some regard,” Fox said.
The other particles experience the Higgs field indirectly. This is possible because quantum mechanical uncertainty allows particles to materialize for brief moments. These fleeting apparitions form clouds of “virtual” particles around more permanent entities. When virtual top quarks crowd around a (second-generation) muon, for example, they could expose the muon to the Higgs field by means of a mutual interaction with a new theoretical particle, giving the muon a bit of mass. But because the exposure is indirect, the particle stays much lighter than the top.
A second round of this game of quantum telephone makes the first-generation electron lighter again by a similar factor, explaining the rough generational spacing of thousands, hundreds, and a few MeV of mass. (The lightest particles of all, neutrinos, also come in three generations. But they act so differently from the other fundamental massive particles that they don’t fit into such schemes.)
Weinberg’s publication considers a whole variety of ways this telephone game could work. He grants the ability to feel the Higgs field to the entire third generation of matter particles—that is, the top quark, bottom quark and tau particle. Mass trickles down to the second and first generations from there via interactions with exotic virtual particles.
Weinberg’s and Fox and Dobrescu’s attempts both fall short, however. The latter two ended up increasing (rather than decreasing) the number of unexplained constants in the Standard Model in order to account for the three-generation particle masses. Weinberg’s proposal gets the relationships between certain masses wrong and fails to describe how higher-generation particles can transform into lower-generation ones (the phenomenon that explains why we don’t see atoms made of second- or third-generation particles). Weinberg was not available to discuss his work, but Fox suggests that Weinberg likely wrote the paper to encourage newcomers to take up the challenge and to flag the problems they’re bound to run into.
Fox sees these hurdles not as fatal blows, but as signs that the theories need more tweaking. “Nature is never exactly how you imagine it at first pass,” he said. “You have some beautiful idea and it sort of gets you 80% of the way there.”
Others aren’t convinced that singling out the third generation and massaging temporary clouds of particles is the right path in the first place. “It seems rather ad hoc because it’s something you put in by hand,” Chen said. She hopes to explain the three generations by embedding the Standard Model within a larger framework like string theory. One model she studies reduces the number of fundamental mass values by adding several new Higgs-like fields to the universe, although the exotic particles associated with these hypothesized fields are far too heavy to search for with Europe’s Large Hadron Collider.
The only solid evidence that could support or distinguish between theories of the matter particles’ masses would be the discovery of the various exotic particles each predicts. The Large Hadron Collider hasn’t seen any, but Fox hasn’t entirely lost hope that the phantasms could someday show up. He believes that experiments probing rare particle transformations, such as the muon-to-electron decay that Fermilab’s Mu2e experiment will study when it goes online this year, have the best chance of indirectly detecting the meddling particles and shaking the Standard Model.
“We don’t know if any of this makes sense,” he said. “We’ll have to wait and see.”
Lead image: Puzzlingly, the laws of nature appear to be composed in triplicate, with three copies of all matter particles, each heavier than the last but otherwise identical. Credit: Lucy Reading-Ikkanda/Quanta Magazine