Snuffling through the underbrush, the shaggy little creature wanders through the sylvan night, sticking its nose in one place, then another, seeking the aroma of its soft-bodied dinner. The forest is dark and the pixie’s eyesight poor, but long whiskers and a keen sense of smell allow it to get around. Threatened, it takes off at breakneck speed, barreling through the vegetation, ducking through holes, soon lost from sight.
An entirely unexceptional lifestyle. Many animals spend their nights cruising the forest floor, searching for small prey in a similar fashion: Hedgehogs, shrews, weasels, to name a few, and bigger ones, too, like opossums and even pigs. The world is full of them.
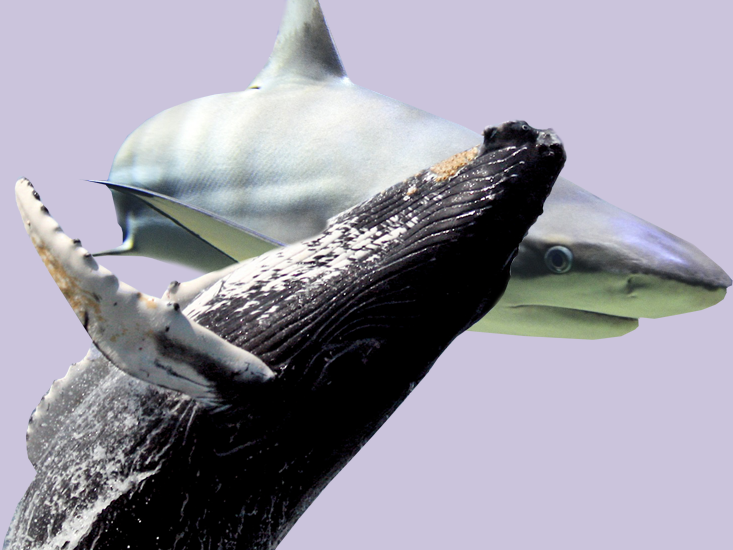
But this one is different. All the others are hairy. This one’s pelage is also soft, made up of millions of thin strands. But they’re not hair. All the others move about on four legs and bear live young. Not this one.
Scratching, probing, sniffing, the animal often duets with its mate, calling back and forth, remaining in contact as they traverse their territory. And as the male calls, he identifies himself: “Kee-wee, kee-wee.”
We’re in New Zealand, and this nocturnal insectivore is a bird, one with nubbins for wings, catlike whiskers, soft feathers, and, unlike any other bird, nostrils on the tip of its beak. Many refer to it as an “honorary mammal.”
New Zealand is chockfull of unusual species. What is equally unusual, however, is what isn’t there: Mammals. There’s scarcely a patch of fur on the islands. Not counting the seals that haul out on New Zealand’s lovely beaches, the only native mammals are a trio of bat species, and even these are weird.
Islands provide a grand cookbook of evolution.
Halfway around the globe, Cuba has its own peculiarities. The owl that was as tall as a first-grader and that may have eaten juvenile giant ground sloths is sadly gone (as are the sloths, one species as big as a gorilla), but the island is still home to a hummingbird as small as a bumblebee; the solenodon, an archaic mammal straight off the pages of Dr. Seuss, with venomous saliva and a long, flexible, bewhiskered schnoz; and beagle-sized guinea pig look-alikes that climb trees and produce copious, banana-shaped, green poops.
Even tiny islands have their unusual curiosities. Lord Howe Island, a five-and-a-half-square-mile crescent lying in the Tasman Sea, is home to six-inch-long black “tree lobsters” that, moniker notwithstanding, are bulky, oversized members of the usually wispy stick insect family. The Solomon Islands in the South Pacific harbor a lizard doing a monkey imitation—the prehensile-tailed skink is a shiny, slender, two-and-a-half-foot-long lizard with a grasping tail that it uses to secure itself as it explores the forest canopy in search of fruit. And everyone’s heard of the erstwhile dodo on the Indian Ocean island of Mauritius, a flightless, fearless, fruit-gobbling pigeon the size of a tom turkey, three feet tall and weighing 40 pounds.
Among small islands, however, the Hawaiian Islands take the prize for evolutionary oddballs: Damselflies whose normally aquatic larvae live on land, voracious carnivorous caterpillars, fruit flies that have deserted their normal fruity fare for decaying plant matter, and other fruit flies that have hammer-shaped heads and defend their territories by headbutting as if they were bighorn sheep. The Hawaiian plant world is equally off-kilter, headlined by the ālula, which looks “like a bowling pin surmounted by a head of lettuce.”
And then there’s Madagascar, sometimes called the eighth continent for the distinctiveness of its biota. There’s a dwarf hippo; an adaptive radiation of lemurs (including a 75-pounder that apparently hung upside down like a sloth and another that looked like a supersized koala, both extirpated by the early human colonists of Madagascar in the last two millennia); ten-foot-tall, half-ton elephant birds (the heaviest birds ever to have lived); half of the world’s species of chameleon, which propel their sticky tongues twice their body length to snare unsuspecting insect prey; fossil frogs the size of an extra-large pizza; crocodiles that were vegetarians; a beetle with a giraffine neck. And the plants of Madagascar are no less unusual, including desert forests composed of tall, slender, spine-encrusted stalks and the stout baobab tree, which looks like it’s been stuck into the ground upside down with roots coming out on top.
Last, but certainly not least, are the wonders of Australia: The duckbilled platypus, kangaroo, and koala, unmatched by anything anywhere else in the world.
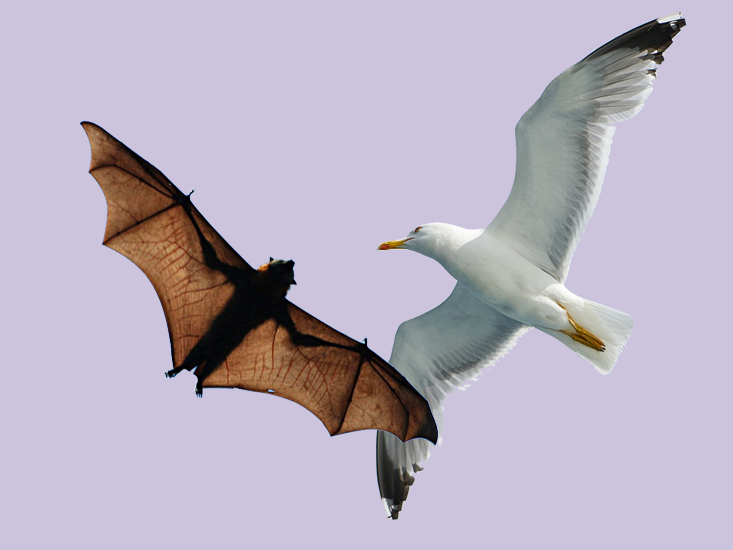
What do all these island oddities add up to? Islands reveal a glimpse of evolutionary alternative worlds, worlds that might have resulted if life had taken a different turn. What if mammals had been wiped out at the end of the Cretaceous along with the dinosaurs? New Zealand gives a suggestion of what might have been. Where would primate evolution have led if monkeys and apes hadn’t evolved? Look no further than the diversity of lemurs, found nowhere else but on Madagascar.
Islands provide a grand cookbook of evolution. And the resulting concoctions inform us that there’s no telling what will come out of the oven. Change the ingredients or the order in which they’re added, turn up the heat, leave something out, use one pinch of salt instead of two, and the result may taste very different. The island cookbook is replete with tales of contingency and chance, the diversity of outcomes suggesting that predicting what will evolve on any given island is very difficult.
For many decades, the accepted narrative in evolutionary biology, as formulated by Stephen Jay Gould, was one of such contingency: Change any one event in the history of life, and all life might have looked entirely different. Life as it exists today was not inevitable or even likely, but a roll of the evolutionary dice.
In recent years, however, a cadre of scientists, led by Simon Conway Morris, has taken the opposite view, arguing that certain evolutionary solutions are indeed quite likely—as evidenced when entirely different species repeatedly evolve the same adaptive solutions to problems posed by the environment—such as the very similar eye structure of humans and octopuses. These repeated solutions are called evolutionary convergence. In this view, the contingencies of history play a minor role, their effects erased by the predictable push of natural selection.
We can easily understand convergence, species adapting in the same way to similar circumstances. But what about the evolutionary one-offs? Why haven’t other species convergently evolved similar adaptations to them?
One explanation for evolutionary one-offs is that these species occur in unique environments. Perhaps they have no analogs because no other species has experienced a similar environment. This, possibly, explains the koala. Its entire lifestyle revolves around living in eucalyptus trees and eating their leaves, which are loaded with poisonous compounds. As a result, the koala’s digestive system is extremely long, providing ample time to slowly detoxify the leaves and extract the nutrients. This slow passage, combined with the low nutrient value of the leaves, means that koalas are on a tight budget, and as a result they minimize energy expenditures, sleeping away most of the day. Eucalyptus trees naturally occur only in Australia, so maybe the singularity of the koala reflects the uniqueness of its environment.
But I suspect this is not the explanation in most cases. Platypuses occur in the streams, ponds, lakes, and rivers of eastern Australia, where they eat crayfish and other aquatic invertebrates that they locate by rooting around on the bottom, sensing their prey with electroreceptors located on their bills. When they’re not out paddling around, they retire to their rest chambers at the end of long burrows dug into the stream bank. The platypus lifestyle would seem to be possible in many places beside Australia. The streams they occupy are much like the creek that ran behind my friend’s house when I was growing up in Saint Louis. Certainly, North America is full of crayfish-packed streams, many in areas with climates similar to the one the platypus experiences, and seemingly with no worse predators than in Australian waterways. So where’s our platypus doppelgänger? Why hasn’t anything like the platypus evolved anywhere else? Or the kangaroo, or any of the other examples I listed, all of which occupy habitats that occur elsewhere?
The other explanation for evolutionary one-offs is that natural selection is either not as predictable or as powerful as some make it out to be. That is, even when species experience identical environments, they might not evolve in the same way.
A key reason for lack of convergence is that there may be more than one way to adapt to a problem posed by the environment. Think about the way vertebrate animals swim. Many use their tail for thrust, but not all tails are the same. Fish tails are vertically flattened and are moved back and forth. Crocodiles swim in the same way. But whale tails are horizontally flattened and are moved up and down. Other animals, like eels and sea snakes, undulate their entire bodies. A few birds, such as cormorants and loons, can move speedily underwater by paddling ferociously with their web-footed hindlimbs. On the other hand, some species swim using modified forelimbs, like the flippers of sea lions and the wings of penguins. However, the most surprising swimmer may be the tree sloth, whose long forelimbs, evolved as an adaptation for hanging upside down, can produce a passing imitation of the Australian crawl. Invertebrates offer even more means of rapid aquatic locomotion, such as the jet propulsion of octopuses and squid.
Maybe the singularity of the koala reflects the uniqueness of its environment.
This list of different ways to move quickly through water brings up the obvious question: To be considered convergent, how similar do the traits of two species have to be? Squid and dolphins use very different anatomical structures to move rapidly through the water—there’s no question that they are not convergent. The foot-propelled locomotion of some aquatic birds is yet another non-convergent means of rapid underwater propulsion.
Other examples, however, are not so clear-cut. What about the tail flukes of cetaceans and sharks, similar in design and operation, but one horizontal and moving up and down, the other vertical and swept left and right? Do these features represent slight variations on a convergent theme, or non-convergent solutions producing the same functional outcome? I suspect that most people would consider horizontal and vertical tail flukes to be fundamentally the same solution.
Let’s move back a step, to a trait that produces the same functional result, but exhibits greater anatomical variation among species. Powered flight evolved three times in vertebrates: in bats, birds, and pterosaurs (the large reptiles that conquered the sky during the Age of Dinosaurs). All three modified their forearms into wings and fly—or flew in the case of pterosaurs—in fundamentally the same way, by flapping a lightweight structure downward to produce lift and forward thrust.
But closer examination reveals that the wings of these flying vertebrates are built in very different ways. The most obvious difference is the aerodynamic surface itself. Birds use feathers, individually growing from the arm bones. In contrast, the airfoil of bats and pterosaurs consists of thin, but very strong skin stretched between finger bones and the body, in some cases even attaching to the hindlegs. The skeletal anatomy of the wings of these three groups of fliers is also very different.
So, are the forearm-modified wings of birds, bats, and pterosaurs convergent adaptations for powered flight that are built in different ways? Or do they represent alternative, non-convergent means of evolving powered flight?
One more example. The largest fish in the sea is the 60-foot-plus whale shark. Like the great whales, it is a filter feeder, gulping enormous quantities of water into its massive mouth and filtering out the minute food upon which it feeds. But that’s where the resemblance ends. The baleenwhales—blue, humpback, gray, and others—strain their prey by pushing water through stiff plates of comb-like baleen that form a curtain hanging from their upper jaws. Any food particle larger than the tiny gaps in the baleen is trapped on the inner surface of the baleen curtain and then ingested. By contrast, whale sharks filter their food in a very different way. Water is pushed out through gill slits positioned on either side at the back of the head. Filter pads made of cartilage are positioned in such a way that the water rushes between the pads, through the gills, and out into the ocean, but food particles continue moving backward past the gill slits, forming a mass in the throat that is subsequently swallowed. So, baleen whales and whale sharks are both large aquatic creatures that use enormous mouths to take in water and filter out small prey. Yet, the precise structure that does the filtering is built, placed, and functions differently. Are these convergent or non-convergent adaptations for filter feeding?
Where one draws the line between convergence and non-convergence among structures that are grossly similar and produce the same functional advantage is arbitrary. My inclination is to consider the wings of birds, bats, and pterosaurs to be convergent. Similarly, I view baleen whales and whale sharks convergent overall because both are large-mouthed, filter-feeding planktivores; however, I consider their filter-feeding structures to be non-convergent, alternative adaptations for filter feeding. But, really, there is no right or wrong answer in cases like these.
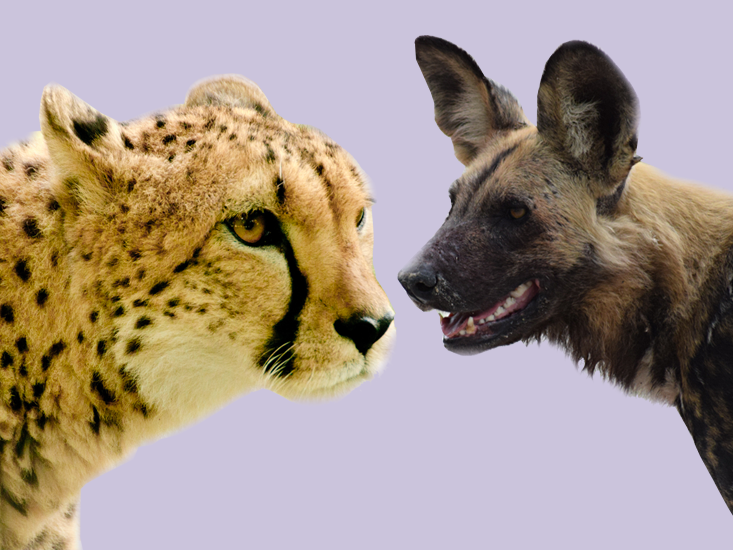
In other cases, though, species can adapt by evolving clearly different, non-convergent phenotypes that produce the same functional capabilities. My favorite example of this phenomenon concerns the subterranean lifestyle of rodents. More than 250 species in the rat clan spend much of their lives underground, moving through self-constructed tunnels. Such burrowing behavior has evolved repeatedly in the Rodentia, but it has been accomplished in different ways. Many rodents dig in the standard way, using their forelimbs to loosen dirt and throw it behind them. The forelimbs of such species are stout and highly muscular; the claws long and strong. Other species use their teeth rather than their claws for soil removal. As you might expect, the teeth of these species are long and protruding, even by rodential standards, and the jaw muscles and skulls are massively constructed. Most dentition diggers get rid of the soil by kicking it backward with their forelimbs, but yet another variation occurs in some rodent species, which pack the loosened soil into the tunnel wall with upward thrusts of their elongated, spade-like snouts. The diverse anatomies of these diggers are a clear illustration of non-convergent adaptations that produce the same functional outcome.
Non-convergence can result for another reason. Often there are different functional ways to adapt to an environmental condition. As an example, consider how potential prey species may adapt to the presence of a predator such as lions. One option is to evolve great sprinting ability to outrun them, but there are other options, too, like camouflage, passive defense, or active defense. The resulting adaptations are decidedly non-convergent, encompassing the horns of the cape buffalo, the body armor of the pangolin and tortoise, the long legs of the impala, the spines of the porcupine, the venom and precision projection of the spitting cobra, and the dappled pelage of the bushbuck.
Multiple solutions to the same selective problem are not limited to defense. Cheetahs and African wild dogs hunt the same prey, but the cat does so by short bursts of great speed, whereas wild dogs run more slowly, but for long periods, exhausting their prey and eventually bringing them down. The adaptations of the two are correspondingly different: The extremely long legs and flexible spine of the cheetah allow it to attain speeds of 70 miles per hour; the great stamina of wild dogs allows them to maintain a steady pace of 30 miles per hour for long enough to fatigue their prey (cheetahs can only sustain their sprints for a short distance).
Or consider the adaptations animals have to obtain nectar. Plants produce the often sweet-smelling, sugary liquid to bribe insects, birds, and other animals to aid in their reproductive process. When an animal sticks its head or entire body into the flower to lap up the nectar, it gets covered with pollen. When the animal goes to the next flower, some of the pollen falls off and fertilizes the plant’s ovules.
Many flowers have very long tubes with the nectar at the bottom—in this way, the plant can limit who gets the pollen to one or a few particular species that are well adapted to using that plant, such as moths with their long proboscises and hummingbirds with similarly long beaks and tongues. Such species, because of their adaptations, probably don’t visit many other types of flowers, limiting the extent that the pollen will fall off in a plant of a different species and thus be wasted.
To be considered convergent, how similar do the traits of two species have to be?
But not all nectarivores play by the rules. Some species of insects, birds, and mammals chew a hole in the base of the flower, bypassing the petals and their pollen and consequently not upholding their end of the coevolutionary bargain. To do so, these nectar thieves possess very different adaptations. Rather than long tongues and mouthparts needed to get to the bottom of long tubes, these species evolve features that enhance their ability to tear through the flower wall. Some hummingbirds have serrated edges on their bills for this purpose; the bird aptly named the flowerpiercer has a sharp hook on the tip of its upper bill used to slice through flowers. What we see in these many examples is that there are often multiple evolutionary options to respond to a challenge posed by the environment. But just because there are multiple possibilities doesn’t mean that all, or even more than one, will evolve. Conway Morris and crew argue that usually one option is superior to the others, and that is why the same trait evolves convergently, time and time again. Yet, convergence doesn’t always occur. Why wouldn’t natural selection favor the same trait every time?
It may be that two (or more) traits are equivalent. Being camouflaged or fleeing at top speed may be equally successful means of eluding predators. Or maybe one approach is more successful than another for a particular purpose, but with other costs that counterbalance its advantage. Rapidly fleeing from an approaching predator may be a better means of escape, but being camouflaged may enhance the ability of an animal like a snake to ambush its own prey. When survival and reproduction are totaled, individuals that are camouflaged may be just as successful as those that rely on speed in reproducing and passing their genes on to the next generation. As a result, natural selection would not necessarily favor one over the other. Which trait evolves might be a matter of chance, a function of which mutation occurs first in the population once it is subject to predation.
Alternatively, which trait evolves might be contingent on the initial phenotype and genotype of the species. A species that was generally active might be predisposed to evolve whatever traits produce greater speed when faced with a new predator, whereas a more sedentary species might instead evolve camouflage. Neither option is superior to the other, but the evolutionary outcome might strongly depend on the initial conditions.
It may also be that one solution actually is superior, but in some cases it’s easier to evolve a suboptimal solution. The French scientist François Jacob, who received the Nobel Prize for his research on how DNA works, proposed an analogy to explain why natural selection would not always lead to the evolution of a perfectly designed organism. Natural selection, Jacob said, is not like an engineer, constructing the optimal solution to the problem at hand. Rather, he said, think about a tinkerer, a handyman who makes use of whatever materials are available to fashion whatever solution is feasible—not the best solution possible, but the best attainable under the circumstances.
Now think of a bird species that finds itself in an area with a lake full of slow fish. It may start diving into the water for a piscine meal and in time may begin to adapt to a more aquatic existence, evolving extra-large and powerful hindfeet like a cormorant or sculpting its wings into flippers à la penguins. Let’s suppose that the best way to swim quickly and with agility is to power through the water with a strong, muscular tail, pushing back and forth or up and down—that’s what the fastest swimmers do. But birds don’t have long tails—they lost them early in their evolutionary history, more than one hundred million years ago, leaving only a tiny residuum of fused bones (the “tails” of birds are composed only of feathers, not bone). I’m not saying that re-evolving a long tail is impossible, but natural selection, the tinkerer, probably wouldn’t take that route. The bird already has wings and feet that can provide some propulsive force. It seems much more likely that natural selection would work to enhance the swimming performance of these pre-existing structures than to evolve a new structure completely from scratch, even if ultimately a remodeled bird with a bony tail—looking something like a cross between a loon and a crocodile—might have been a better swimmer.
But, still, if a bird-crocodile would be better adapted—a superior, faster swimmer—why wouldn’t the swimming bird continue to evolve in that direction? The answer may be that sometimes you can’t get there from here: evolving from one adaptive form to another may be difficult because intermediate conditions are inferior. A long, powerful tail may be great for rapid propulsion, but a short flap of a tail may just get in the way, actually decreasing swimming performance. Natural selection has no foresight—it won’t favor a detrimental feature just because it is an early step on a path leading to an ultimately superior condition. Rather, for a feature to evolve by natural selection, every step along the way must be an improvement on what came before it—natural selection will never favor a worse condition, even if it’s only a transient evolutionary phase.
So, where does that leave us? Is convergence pervasive, a demonstration of inherent structure in the biological world, channeled by predictable forces of natural selection toward outcomes predestined by the environment? Or are examples of convergent evolution the exceptions, cherry-picked illustrations of biological predictability in a haphazard world in which most species have no evolutionary parallels?
We could argue these points back and forth until we’re blue in the face. I’d throw out the platypus, you’d counter with convergent hedgehogs; I’d postulate the unique, algae-encrusted, upside-down-hanging tree sloth, you’d retort with bipedal-hopping mice independently evolved on three continents. And that is how, essentially, this controversy has been debated historically, by compiling lists and telling stories.
Conway Morris and his colleagues are to be commended for bringing convergent evolution to the forefront. We all knew about convergence as a neat trick of natural history, a striking example of the power of natural selection. But Conway Morris and company have made clear that evolutionary duplication is much, much more common than we realized. We now recognize that it’s a frequent occurrence in the natural world, with examples all around us. Still, it’s far from ubiquitous.
Seemingly just as often, maybe more often, species living in similar environments don’t adapt convergently. At this point, we need to go beyond documenting the historical pattern, chronicling yet more examples pro and con. Rather, we need to ask whether we can understand why convergence occurs in some cases and not others—what explains the extent to which convergence does or doesn’t occur, why bipedal-hopping rodents have evolved independently in deserts around the world, but the kangaroo has only evolved once. And to do that, we need to do more than add additional examples to our lists. We need to test the evolutionary determinism hypothesis directly.
Evolutionary biology was late to the experimental game—evolution’s legendarily languid pace made the idea of experiments a non-starter. We now know that this view is mistaken, that evolution can proceed very quickly. And that realization opens a new door to the study of evolution.
Jonathan B. Losos is a biology professor and director of the Losos Laboratory at Harvard University and Curator of Herpetology at Harvard’s Museum of Comparative Zoology. He is the author of Lizards in an Evolutionary Tree: Ecology and Adaptive Radiation of Anoles.
From Improbable Destinies: Fate, Chance, and the Future of Evolution by Jonathan B. Losos, published by Riverhead Books, an imprint of Penguin Publishing Group, a division of Penguin Random House LLC. Copyright © 2017 by Jonathan B. Losos.
Lead Image Credit: Leonello Calvetti / Getty Images