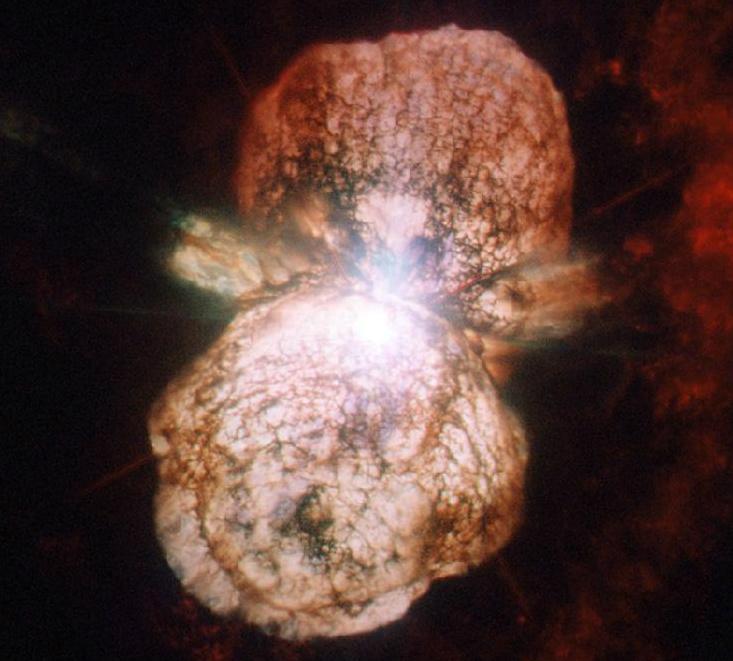
When our Universe was in its infancy, the only element it contained was hydrogen, the simplest one—not nearly enough by itself to create interesting things like planets and people. By the time things cooled sufficiently for the single proton in each hydrogen atom to pair with a negatively charged electron, about 92 percent of the atoms in the Universe were hydrogen. The rest had fused mostly into helium with traces of lithium and a few other light elements. At that time the Universe was too cold for other elements to form, and the cosmos entered a dark age lasting 380 million years.
As the Universe expanded and cooled, gravity began to take hold. Galaxies coalesced, and soon the first stars began to shine. At first this light was only due to the weight of a star itself: As a star collapses under its own weight, the hydrogen is squeezed together, which causes it to heat up, the way putty warms when you squeeze it in your hand. By itself, gravity could only keep these stars shining for a few million years, but it was enough to heat the cores of these stars just enough for the alchemy of nuclear fusion to begin.
The fact that fusion can occur in this way is in many ways astounding. Fusion is not simply a union of two nuclei. In most stars, hydrogen nuclei can’t get close enough to fuse. The closer a pair of hydrogen nuclei get, the more strongly their positive charges push them apart. But because nuclei are quantum objects, they don’t need to be close enough to fuse, just to be in the same ballpark. From there an effect known as quantum tunneling can do the rest. One moment the two nuclei are almost close enough to fuse, and the next moment they suddenly find themselves bonded together. It is as if the nuclei don’t have enough energy to open the door and walk through, but they occasionally will teleport through walls.
But even this bit of quantum magic isn’t enough for a star to succeed. Not only does fusion have to occur, it has to produce something stable. When two protons fuse to become helium-2 (containing two protons and no neutrons), it is extremely unstable and usually splits right back into two separate protons. But there is a 1 in 10,000 chance that one of the protons will instead transform into a neutron, and the atom then becomes deuterium, a stable isotope of hydrogen. Deuterium and hydrogen can fuse to make a stable helium, releasing a huge amount of energy and opening up the amazing creative potential of stars.
Each step from hydrogen to the other elements relies upon rare celestial and quantum processes. It is a parade of flukes that leads from primordial matter to us.
For small stars, hydrogen is the only element they can fuse; when they run out, they go dark. But after the largest of the first stars transformed their hydrogen to helium, they burned on in another way. When these large stars stopped fusing hydrogen, their internal pressure went down, gravity began to collapse them again, and the temperature of their cores rose. As their cores reached a temperature of a hundred million Kelvin, helium began to fuse into beryllium (an atom with four protons), and beryllium and helium fused to produce carbon (six protons). The element central to life on Earth began to form in the blazing hearts of stars, though this carbon still had a long journey ahead before it would become a part of us. From carbon fusion comes nitrogen and oxygen (seven and eight protons, respectively), two more elements necessary for life, and from these comes a chain of fusion up to iron (26 protons).
Fusing iron into heavier elements doesn’t produce more energy, as the fusion of lighter elements does—when iron fuses, it absorbs energy, which is actually a good thing. If elements always fused into heavier elements, then the first stars would have simply fused indefinitely, until they became neutron stars, enormous, undifferentiated orbs of nuclear material. But because the fusion of iron actually cools the core of a star, the chain of fusion shuts down. After their fusion stopped, the first big stars eventually collapsed under their own weight, which triggered supernova explosions. The outer layers of each star, rich in carbon, nitrogen, and oxygen, were cast into interstellar space, and only the cores of these stars collapsed, yet again, into neutron stars.
It had long been thought that stellar fusion and supernova explosions were enough to account for the diversity of elements we see around us. But we now know that other exotic processes play a key role as well. Recent studies have shown that rare, heavy elements like gold are produced in large quantities when two neutron stars collide. It is likely that all the gold on Earth was produced by such a collision. (See “Earth’s Stash of Gold Comes From Colliders Fit for Gods” for more detail.)
Each step from hydrogen to the other elements relies upon rare celestial and quantum processes. It is a parade of flukes that leads from primordial matter to us. Each link in the chain is not only unlikely, but extremely sensitive to the physics involved. If the strong force binding protons together were just 2 percent stronger, helium-2 would stable (pdf). Fusion would occur much more easily, and the first generation of hot, dense stars would not have emerged. Tweak any part of the process and the Universe would look very different, and we likely wouldn’t exist.
This is sometimes referred to as the fine-tuned Universe, the idea that the existence of life is crucially dependent upon phenomena such as stellar fusion happening in just the right way. It is an idea that has led some to invoke theological arguments about the origin of the Universe, while others argue just the opposite. Either way, one thing is clear: We are the Universe made manifest. Everything we we are and everything we see around us is hydrogen, transformed by the crucible of gravity and time.
Brian Koberlein is an astrophysicist and physics professor at Rochester Institute of Technology. He writes about astronomy and astrophysics on his blog One Universe at a Time. You can also find him on Twitter @BrianKoberlein.