Part of Dennis Plenker’s daily job is growing cancer. And a variety of different ones, too.
Depending on the day and the project, different tumors may burgeon in the petri dishes stocked in the Cold Spring Harbor Laboratory where Plenker works as a research investigator. They might be aggressive breast cancers. They might be glioblastomas, one of the deadliest brain tumors that rob patients of their ability to speak or read as they crowd out normal cells. Or they might be pancreatic cancers, the fast and vicious slayers that can overtake a healthy person within weeks or even days.
These tiny tumor chunks are transparent and bland—they look like little droplets of hair gel that accidentally plopped into a plastic dish and took hold. But their unassuming appearance is deceptive. If they were still in the human bodies they came from, they would be sucking up nutrients, rapidly growing and dodging the immune system defenses. But in Plenker’s hands—or rather in the CSHL’s unique facility—these notorious killers don’t kill anyone. Instead, scientists let them grow to devise the most potent ways to kill them.
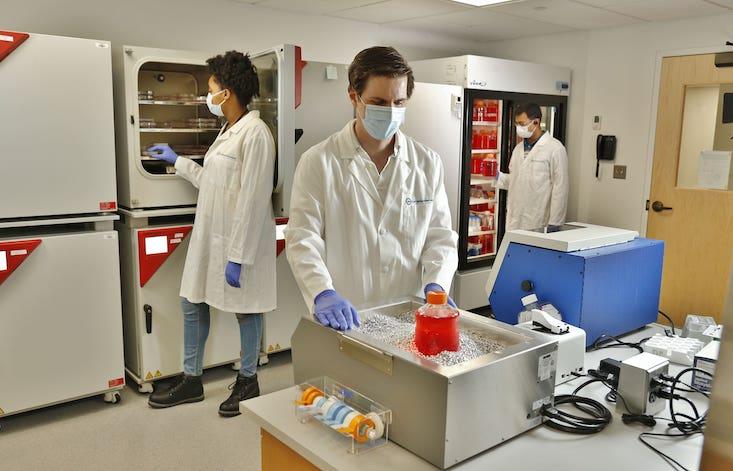
These tumor chunks are called organoids. They are three-dimensional assemblages of malignant growths used to study cancer behavior and vulnerability to chemotherapy and the so-called “targeted drugs”—the next generation therapies. Scientists used to study tumors at a single-cell level, but because tumors grow as cell clusters in the body, it proved to be inefficient. The three-dimensional structures make a difference. For example, chemo might destroy the tumor’s outer cell layer, but the inner ones can develop resistance, so where single cells may die, a 3D mass will bounce back. Organoids can provide a window into these little-known mechanisms of drug resistance. They can reveal how normal tissues turn malignant and where the cellular machinery goes off-track to allow that to happen.
As their name suggests, organoids are scientists’ windows into organs, whether healthy or stricken with disease. You need to know your enemy to beat it, Plenker says, and cancer organoids offer that opportunity. Taken from patients currently undergoing cancer treatments, these tumor chunks will reveal their weaknesses so scientists can find the cancers’ Achilles’ heel and devise personalized treatments. “Organoids are essentially patients in a dish,” Plenker says. Only unlike real patients, the organoids can be subjected to all sorts of harsh experiments to zero in on the precise chemo cocktails that destroy them in the best possible way. And they will likely provide a more realistic scenario than drug tests in mice or rats, as animal models aren’t perfect proxies for humans.
These notorious killers don’t kill anyone. Instead, scientists devise the most potent ways to kill them.
The way that cancer proliferates in the body is hard to reproduce in the lab. Stem-cell research made it possible. After scientists spent a decade understanding how various cells multiply and differentiate into other cell types based on molecular cues and nourishment, they were able to make cells grow and fuse into tissues. To stick together like bricks in a nicely laid wall, cells need a biological scaffold that scientists call an extracellular matrix or ECM, which in the body is made from collagen and other materials. Today, the same collagen scaffolds can be mimicked with a gooey substance called Matrigel—and then seeded with specific cells, which take root and begin to multiply.
Some tissue types were easy to grow—Columbia University scientists grew viable bones as early as 2010.1 Others, like kidney cells, were trickier. They would grow into immature tissues incapable of performing their job of cleaning and filtering blood. It took scientists time to realize that these cells wanted more than scaffolding and food—they needed to “feel at home,” or be in their natural habitat. Kidney cells needed the feeling of liquid being washed over them, the Harvard University group found, when they first managed to grow functioning kidney tissue in 2018.2
Cancers have their own growth requirements. In the body, they manage to co-opt the organism’s resources, but keeping them happy in a dish means catering to their dietary preferences. Different cancers need different types of molecular chow—growth factors, hormones, oxygen and pH levels, and other nutrients. Pancreatic adenocarcinoma thrives in low-oxygen conditions with poor nutrients.3 Glioblastomas feed on fatty acids.4 These nutrients are delivered to organoids via a specific solution called growth medium, which the lab personnel regularly doles out into the dishes.
Plenker is charged with keeping this murderous menagerie alive and well. He is the one who designs the cancers’ dietary menu, a specific protocol for each type. And while his official title is facility manager and research investigator who works closely with David Tuveson, director of the CSHL’s Cancer Center, he is essentially a cancer custodian, a curator of a unique collection that aims to change the paradigm of cancer treatment.
Plenker’s research area is pancreatic cancer—one of the most notorious killers known. Often diagnosed late and resistant to treatment, it is essentially a death sentence—only 8 to 10 percent of patients remain alive five years after diagnosis. The chemo drugs used to treat it haven’t changed in 40 years, Plenker says. In the past decade, physicians tried combining multiple drugs together with relative success. Identifying winning combos can save lives, or at least prolong them—and that’s what the organoids will help clinicians do better.
In a groundbreaking clinical trial called PASS-01 (for Pancreatic Adenocarcinoma Signature Stratification for Treatment), Plenker’s team collaborates with other American and Canadian colleagues to identify the most effective chemo cocktails and to understand the individual patients’ tumor behaviors, which would lead to more personalized treatments.5
Scientists know the same cancer types behave differently in different patients. Typically, all malignancies have the so-called “driver mutation”— the cancer’s main trigger caused by a mutated gene. But tumors also often have “passenger mutations” that happen in nearby genes. These additional mutated genes can generate various proteins, which may interfere with treatment. Or not. Scientists call these mutated gene combinations tumor mutational signatures, which can vary from one patient to the next. With some cancers, doctors already know what mutations signatures they may have, but with pancreatic cancer they don’t have good tale-telling signs, or biomarkers. “There aren’t many biomarkers to help clinicians decide which chemo may be better for which patient,” explains oncologist Grainne O’Kane, who treats pancreatic cancer patients at the Princess Margaret Hospital in Toronto, Canada.
That’s the reason O’Kane participates in the PASS-01 trial—it will give doctors a better view into the exact specifics of their patients’ malignancies. As they take their patients’ biopsies, they are sending little cancer snippets to the CSHL to be grown into organoids, which will be subjected to chemo cocktails of various combinations to design more personalized regiments for them.
The hospital treats all patients with the so-called standard of care chemotherapy, which is more of a one-size-fits-all approach. Some patients will respond to it but others won’t, so the goal is to define the second line of chemo defense in a more personalized fashion. “That’s where the biopsies we send to Tuveson’s lab might be useful,” O’Kane says. “They can help us find something to benefit patients after the first line of chemo stopped working.”
Organoids are patients in a dish. Unlike real patients, organoids can be subjected to experiments.
Scientists can try all kinds of combos on the tumorous organoids, which they can’t do in living people. “You can treat 100 organoids with 100 different compounds and see which one works, or which compound does a good job and which ones don’t work at all,” Plenker says. That would also allow scientists to define the precise amount of chemo, so doctors wouldn’t have to over-treat patients with harsh drugs that create sickening side effects. Ultimately, organoids should take a lot of guesswork out of the process.
With about 150 patients’ adenocarcinomas already collected, the team hopes to come up with some answers. O’Kane says her team already has three patients for which they were able to design the more personalized second line of defense chemo, based on what their organoids revealed. They haven’t yet tried it, because the trial has only started recently, but this would be the next step. “Being able to piece all this information together in real time as patients are moving through their therapies can really improve the outcomes,” O’Kane says. And while they may not be able to save all of those who graciously donated their biopsy snippets to science, it will help build better treatments in the future. “Even if we won’t be able to help these specific patients we’re hoping to use this info in the future clinical trials,” O’Kane says.
Organoids can also help understand how cancer develops. This is particularly true for breast cancers, says Camilla dos Santos, associate professor and a member of the CSHL Cancer Center. She studies the inner life of human mammary glands, more commonly referred to as breasts, and is also part of the cancer custodian crew. The hormonal changes that women go through during pregnancy subsequently modify breast cancer risk, sometimes lowering it and sometimes increasing—a complex interplay of the body’s chemicals.
“We know that women who get pregnant for the first time before they turn 25 years old, have a 30 percent decrease in breast cancer incidents later in life,” dos Santos says. “When they turn 60 or 70, 30 percent of them will not develop cancer.” On the contrary, those who are pregnant past 38 have a 30 to 50 percent increase in developing aggressive breast cancer types. Clearly, some molecular switches are involved, but they are very hard to study within the body. That’s where organoids can provide a window into the surreptitious process.
Using breast organoids, scientists can model the complex life of mammary glands at various stages of a woman’s life. And while most women wouldn’t want their breasts poked and pierced when they are pregnant or breastfeeding, many donate their tissues after breast reduction surgery or prophylactic mastectomy due to high-risk mutations like the BRCA gene. That’s where organoids shine because scientists can not only grow them, but also give them the pregnancy hormonal cues, which will make cells generate milk, stop lactating, or do it again—and study the complex cellular interactions that take place in real life.
There’s a lot to study. At birth, mammary glands are similar in both genders—just little patches of the mammary epithelium tissue. But when puberty hits, the female glands fill up with the so-called mammary tree—a system of ducts for future milk production, which fully “blooms” in pregnancy. “When a woman becomes pregnant, the duct tree expands, growing two types of cells—luminal and myoepithelial ones,” explains Zuzana Koledova, assistant professor of Masaryk University in Czech Republic who also uses organoids in her work. When the baby is born, the luminal cells, which line the inside of the ducts, produce the proteins that comprise milk. The myoepithelial cells reside outside the ducts and work as muscles that squeeze the ducts to push milk out. Dos Santos likens this pregnancy mammary gland growth to the changes of the seasons. The images of sprouting ducts look like blossoming trees in the spring while later they shrivel like plants do in the fall.
The body governs these processes via the molecular machinery of hormones, which stimulate breast cells growth during pregnancy, and later cause them to die out. The two pregnancy-related hormones, prolactin and oxytocin, are responsible for milk production. Prolactin induces the luminal cells to make milk while oxytocin makes the myoepithelial cells contract. Once the baby is weaned, the levels of these hormones drop, causing cells to shrink back to their non-pregnant state.
With organoids scientists can observe these cellular dynamics at work. Koledova’s team had watched breast organoids secrete milk based on biological cues. They even recorded movies of cells pumping tiny milk droplets in the dish they were growing in. Using tiny snippets of donated breast tissue, the team grew the organoids inside the Matrigel matrix in the growth media and then added the two pregnancy hormones into the mix, explains Jakub Sumbal, a mammary gland researcher in Koledova’s group. As they began to secret proteins that compose milk, the organoids, which looked like little domes inside the dish, changed from translucent to opaque. “At first, you can see through them, but then as they produce these proteins, they kind of darken,” Sumbal says. “And you can see them pushing out these little droplets.”
Cancer patients would no longer have to undergo chemotherapy by trial and error.
Dos Santos’s team, who also did similar work, outlined molecular changes that follow such dish-based hormonal cues in their recent study.6 In response to hormonal messages, cells produce proteins, which they display on their surfaces, like status symbols. During pregnancy the burgeoning cells prepping for milk production display the “proteins flags” that make them look important, attracting nourishment. When it’s time to die, they commit a cellular suicide. They signal to the bypassing macrophages—immune system cleanup crew—to devour them. “They essentially say ‘come eat me!’ to the macrophages,” dos Santos says. “Because I’m no longer needed.”
The ability to mimic these processes in a dish, allows scientists to study the molecular switches that trigger breast cancer development—or minimize it. Scientists know that cancerous cells can hide from the immune system and even co-opt it into protecting themselves. They do it by displaying their own “do not eat me” protein flags on the surface and avoid destruction. “Sometimes cancer cells can recruit specific types of immune cells to protect them,” dos Santos says. “They can not only say ‘do not eat me,’ but say ‘come hang out with me’ to the macrophages, and the macrophages will send suppressive signals to the B-cells or T-cells, the body defenders.” It is as if the cancer requests protection—a crew of guardians around it to defend against other cells that would otherwise wipe it out.
Scientists can’t telescope into the body to peek at these interactions, but they now can watch these stealth battles unfolding in a dish. “Right now we are looking at the proteins that are secreted by the organoids—the proteins that go on the surface of the organoids’ cells and what they would communicate to the immune system,” dos Santos says. “Even when there’s no immune system surrounding them, they would still be doing that.” There’s a way to mimic the immune system, too. Scientists can add B-cells, T-cells, macrophages, and other players into the growth medium and watch the full-blown cellular warfare in action. “That’s the next step in our research,” dos Santos says.
Understanding what hormonal fluxes trigger breast cancer, and how it recruits other cells for safekeeping, can give scientists ideas for pharmaceutical intervention. “We can find drugs that pharmacologically turn off the switches that trigger cancer or interrupt its signaling for protection,” dos Santos says. “That opens novel ways to treat people.”
Can organoid research lead to a new standard of care for cancer patients? That’s the ultimate goal, researchers say. That’s why Plenker works at keeping his collection of cancer glops alive and well and thriving—he calls it a living biobank. And he keeps a stash in the cryogenic freezer, too. He is also developing protocols that would allow commercial companies to grow organoids the same way chemical industries make reagents or mice suppliers grow rodents for research. A benefit of organoid experiments is they don’t involve animals at all.
Hospitals may one day start growing organoids from their patients’ biopsies to design and test personalized chemo cocktails for them. Once science crosses over to that reality, the entire treatment paradigm will change. Cancer patients won’t have to undergo chemotherapy by trial and error. Instead their cancer organoids will be subjected to this process—knocked out by a gamut of drug combinations to find the winning one to use in the actual treatment. Plenker notes that once enough data is gathered about the tumors’ mutational signatures, scientists may create a database of tumor “mugshots” matching them to the chemo cocktails that beat them best. And then just sequencing a biopsy sample would immediately inform oncologists what drug combo the patient needs. “We may be about 10 years away from that,” Plenker says, but for now there’s a lot more research to do. And a lot more cancers to grow.
Lina Zeldovich grew up in a family of Russian scientists, listening to bedtime stories about volcanoes, black holes, and intrepid explorers. She has written for The New York Times, Scientific American, Reader’s Digest, and Audubon Magazine, among other publications, and won four awards for covering the science of poop. Her book, The Other Dark Matter: The Science and Business of Turning Waste into Wealth, will be released in October 2021 by Chicago University Press. You can find her at LinaZeldovich.com and @LinaZeldovich.
References
1. Grayson, W.L. et al. Engineering anatomically shaped human bone grafts. Proceedings of the National Academy of Sciences 107, 3299-3304 (2010).
2. Homan, K.A. et al. Flow-enhanced vascularization and maturation of kidney organoids in vitro. Nature Methods 16, 255-262 (2019).
3. Yuen, A. & Diaz, B. The impact of hypoxia in pancreatic cancer invasion and metastasis. Hypoxia (Auckl) 2, 91-106 (2014).
4. Sperry, J. et al. Glioblastoma utilizes fatty acids and ketone bodies for growth allowing progression during ketogenic diet therapy. iScience 23 (2020).
5. Jaffee, E. & Knox, J.J. Pancreatic Adenocarcinoma Signature Stratification for Treatment (PASS-01). Identifier NCT04469556 (2020). https://clinicaltrials.gov/ct2/show/NCT04469556
6. Ciccone, M.F., Trousdell, M.C., & Dos Santos, C.O. Characterization of organoid cultures to study the effects of pregnancy hormones on the epigenome and transcriptional output of mammary epithelial cells. Journal of Mammary Gland Biology and Neoplasia 25, 351-366 (2020).
Lead image: ProStockStudio / Shutterstock
Support for this article was provided by Cold Spring Harbor Laboratory. Read more on the Nautilus channel, Biology + Beyond.