Happiness, in one form or another, seems to be a common goal that most of us would like to attain. We often behave as though we might find a route to contentment—comfort, satiety, warmth, or some other reward—and be happy all the time if we could just make the right choices. But pleasure is often fleeting, even from the most appealing experiences, giving rise to ennui and sparking the drive for something new and sensational. As a neuroscientist, I can’t help wondering whether the transience of our satisfaction may not in fact be inescapable and instead may reveal an inevitable aspect of the way the brain works, the understanding of which might provide a clue to how to contend with it.
Many moment-to-moment functions of the brain seem so natural that we can hardly distance ourselves enough to reflect upon them: The brain notices. It is obvious, once we consider it, that a basic job of the brain is to perceive; with those perceptions it can evaluate; and based on those evaluations, it can act. This work is carried out by neurons of the nervous system. They detect and represent input from the outside world (and the inside world), analyze the data, and then respond to this analysis with an appropriate action. Action generally involves movement: Neurons send the signals that make muscles contract and let you do things. The input is sensory, the analysis is often called associative, and the output is motor. The sensory-associative-motor triplet is the neural version of perceiving, evaluating, and doing.
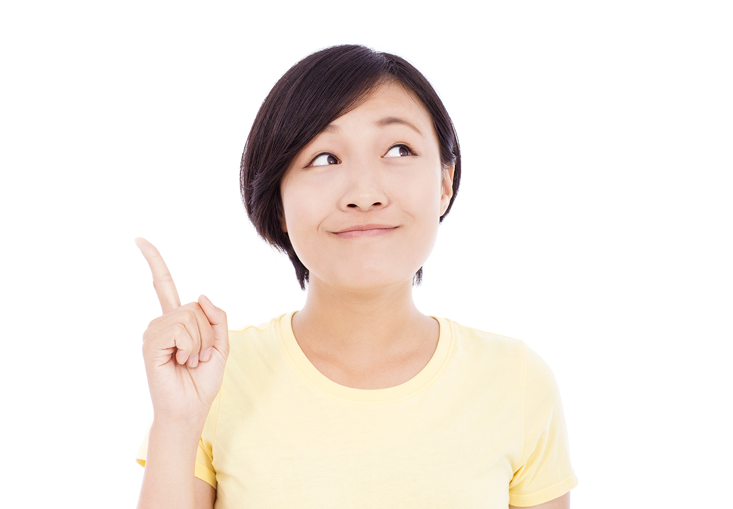
How do the neurons that compose the brain conduct the business of detecting and analyzing what is happening out there in the world? The simplified answer is that they rely first on a translation service. The body parts that we think of as our sense organs—eyes, ears, noses, tongues, skin—contain sensory receptor cells, so called because they receive information. Tiny protein molecules sit on the membranes of those cells and translate (or, to use the technical term, transduce) physical stimuli from the outside world—light, sound, chemicals, and heat—into electrical signals called action potentials, which form the language of the brain. The transduction proteins form or connect to a minuscule pathway, or ion channel, through which charged particles called ions, like sodium and potassium, enter or exit the cell. The movement of ions makes the electrical signals. Each electrical signal spreads along the length of the cell by means of other proteins—which also form ion channels—ultimately culminating in the release of a chemical neurotransmitter. The next neuron receives the neurotransmitter via other receptor proteins, which also are themselves ion channels or are coupled to ion channels. Our ability to notice lies largely in our ion channel proteins.
We perceive things not by their absolute value but by their contrast to what came before.
The interesting part is that nearly all these proteins respond to changes in stimuli, but in the presence of long-lasting, constant stimulation of mild to moderate intensity, many of them quite literally shut themselves down and stop allowing ions to flow through them. We call this process adaptation (or desensitization or inactivation, depending on its physical basis). It leads to familiar experiences with respect to the senses. Adaptation is why, for example, when you go from a brightly lit space into a dim room, it seems dark at first, but after a while you no longer perceive darkness; the lighting seems normal. Only when you go back into the sun does the change make you realize how dim it was before—or how brilliant it is now. Similarly, most people adapt to the scent of cooking soon after entering a restaurant, or the coolness of a swimming pool after jumping into it on a hot day, or the background hum of a refrigerator. After a short exposure, the aroma or chill or noise—unless it is overwhelming to the point of discomfort—becomes undetectable and goes unnoticed. In the common parlance, you get used to it. In part because of our adapting ion channels, we perceive many things not by their absolute value but by their contrast to what came before.1 In an extreme case, experimentalists have been able to demonstrate this phenomenon by stabilizing an image on the retina. Our eyes generally dart around in so-called microsaccades, which let our retinal cells compare the light reflected from dark and light areas in any visual scene. By monitoring a person’s eye movements and shifting a projected image accordingly, visual neuroscientists can demonstrate that when an image is artificially held in a fixed position on the retina, the person “sees” the image disappear.2 Without one’s being able to make comparisons, the world goes gray. In other words, it’s not just that variety is the spice of life; it’s variance that lets us sense anything at all.
This sensitivity to change and insensitivity to constancy doesn’t stop at the level of sensory receptors. Deeper in the brain, in almost every neuron, are other ion channel proteins—particularly sodium channels, which start action potentials (by letting sodium ions into a neuron), and potassium channels, which end action potentials (by letting potassium ions out of a neuron). Both sodium and potassium channels come in many varieties, and many of these ion channels also inactivate—that is, turn themselves off—with use. Consequently, even when chemical neurotransmitters provide a prolonged or repeated stimulus to neurons, the intrinsic properties of ion channels limit how many action potentials are produced. For instance, in some neurons, the inactivation of sodium channels makes it progressively more difficult for action potentials to be generated in the face of constant stimulation.3 Meanwhile, specific potassium channels gradually increase their ion flow, helping to slow or shut down a neuron’s signaling after a few action potentials. This interaction between sodium and potassium ion flow permits electrical signals to be generated only at the outset of a stimulus, a process called accommodation. Although there are exceptions, most of the principal excitatory cells of the cortex and hippocampus—the ones that encourage action potentials in the neurons they target—tend to accommodate.4 We don’t always know what kinds of information these accommodating neurons are carrying, but we do know that they respond most strongly to changing stimuli.5
Similarly, neurotransmitter receptor proteins can undergo desensitization, in which their ion channels shut themselves off in real time as prolonged stimuli arrive at the neuron.6 But neurons also have an interesting ability to respond to long-term increases in neurotransmitter exposure—over days or longer timeframes which might result from excessive signaling through a particular neural circuit—by simply consuming their own neurotransmitter receptors, leaving fewer working receptors available on the cell surface. In part, such responses can underlie tolerance to medications, drugs of abuse, and even spicy food.7 Conversely, when neurotransmitter release falls, a given neuron can produce more receptor proteins and associated ion channels. In this way, overstimulation reverts to producing a normal degree of input, and understimulation sets up a neural circuit to be extra sensitive even to small signals. How does a cell know? A variety of cellular feedback systems, many of which make use of the special biochemical properties of calcium ions, allows neurons to figure out, so to speak, the comfortable or appropriate point between too much and too little. Processes like these may be engaged when a stimulus that was initially pleasurable—or aversive—is experienced over and over again. The acute perception fades as the brain finds its own set point.8
At the level of the whole organism, the feelings from those perceptions fluctuate accordingly, diminishing to repeated stimuli and being restored only when a change takes place. A simple illustration of this phenomenon occurs in the sea slug Aplysia, which initially retracts its gill in response to a light touch. With a series of harmless touches, it habituates, ceasing to react, until the touch is paired with something more aversive, like a shock.9 In a more pleasurable arena of sensation, hungry rats will expend effort to obtain either ordinary or palatable food, whereas rats that have eaten to satiety will work only to get new treats they find especially tasty. The rats’ motivation to work for edibles can be reduced by drugs that interfere with receptors for natural opiates and dopamine, which are neurotransmitters in brain circuits that signal rewards. The idea, therefore, is that reward pathways are stimulated by the anticipation and/or consumption of food, but for sated rats only if the food compares favorably with their recent experience.10 In other words, there is no need to save room for dessert; it will be just as pleasurable as long as it is better than what came before.
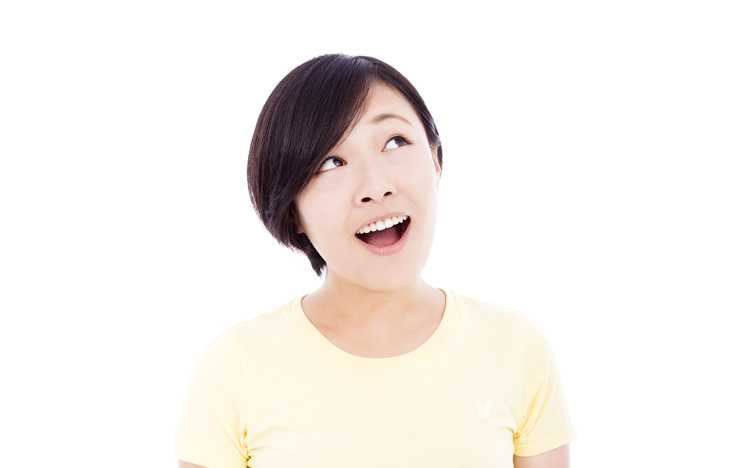
Familiar stimuli and the experiences they generate can also trigger other modifications of ion channels and neurotransmitter receptors, and these modifications can alter whole neural circuits. In fact, certain circuits in the brains of many animals (including us) are so good at predicting the outcome of well-known stimuli that they send inverse signals that actively cancel out the perception of what is going on. The organism doesn’t even notice what is happening—at least until something different or surprising intervenes.11 The ability to get used to and ultimately ignore incoming information that is static, familiar, predictable, and non-harmful turns out to be helpful behaviorally; in other words, it offers an evolutionary advantage. Continuing to notice sensations like the light touch of our clothes on our arms or the mild fragrance of the laundry detergent we used to wash them would be distracting, to say the least, and might even interfere with our ability to detect and respond to a signal that mattered, like a tap on the shoulder or our toast burning. In fact, an inability to predict and thereby adapt may be a contributing factor to conditions like autism spectrum disorders.12 Besides, it’s wasteful to send brain signals to report information we already know about. When all those ions flow in and out of cells to send signals within our brains, they cannot just remain on the opposite side from where they started. It literally consumes energy to pump sodium back out of neurons and potassium back into them, so it is most efficient not to generate action potentials that don’t carry worthwhile information.
Does this mean that only novelty matters and that everything familiar must be discarded once the experience wears off? On the contrary; I think it offers a key to happiness that is compatible with how the brain works. The ability to detect even familiar stimuli can usually be restored by a brief palate cleanser, which literally permits a recovery from desensitization sufficient to intensify a subsequent experience. It is hard for me to assess how much I am waxing poetic, but it seems to me that the brain’s ability—its need—to perceive by contrast may partly explain why our efforts to achieve perennial satisfaction have been largely unsatisfactory. Because the brain grades on a curve, endlessly comparing the present with what came just before, the secret to happiness may be unhappiness. Not unmitigated unhappiness, of course, but the transient chill that lets us feel warmth, the sensation of hunger that makes satiety so welcome, the period of near-despair that catapults us into the astonishing experience of triumph. The route to contentment is through contrast.
Indira M. Raman is a professor in the department of neurobiology at Northwestern University.
By Indira M. Raman, as published in Think Tank: Forty Scientists Explore the Biological Roots of Human Experience, edited by David J. Linden, and published by Yale University Press.
Footnotes
1. Not all behavioral adaptation comes from ion channels that change rapidly, on the timescale of the stimulus. Many other long-term changes can take place, some of which may involve ion channel function and some of which tap into a variety of cellular processes. Also, not every sensation or perception is subject to adaptation, one of the most familiar sensations being the experience of pain.
2. Ditchburn, R.W. & Ginsborg, B.L. Vision with a stabilized retinal image. Nature 170, 36-37 (1952).; Martinez-Conde, S., Macknic, S.L., Troncoso, X.G., & Dyar, T.A. Microsaccades counteract visual fading during fixation. Neuron, 49, 297-305 (2006).
3. In contrast, some neurons have specialized sodium channels in which inactivation is circumvented by the intervention of an additional protein that literally blocks the usual inactivation process. These neurons readily fire long trains of high-frequency action potentials. Many such neurons are found in the cerebellum and brainstem. Lewis, A.H. & Raman, I.M. Resurgent current of voltage-gated Na+ channels. Journal of Physiology 592, 4825–4838 (2014).
4. In some cells, accommodation can be reversed by neurotransmitters like noradrenaline (norepinephrine), which suppresses the ionic current through specific potassium channels, called SK channels. It is interesting that the global effect on the brain of noradrenaline is often an increase in attention. Many toxins and poisons, such as those of scorpions and snakes, however, also prevent inactivation of sodium channels and block potassium channels, leading to convulsions and death, again revealing that the brain can suffer from too much of a good thing. Madison, D.V. & Nicoll, R.A. Actions of noradrenaline recorded intracellularly in rat hippocampal CA1 pyramidal neurones, in vitro. Journal of Physiology 372, 221–244 (1986).; Hille, B. A K+ channel worthy of attention. Science 273, 1677 (1996).
5. Although it is hard to resist the tempting conclusion that more brain activity is necessarily better, the ability of some neurons to shut down their own neuronal signaling by ion channel inactivation is often a good idea. A variety of neurological diseases is associated with too many action potentials in neurons that normally signal relatively sparsely. These disorders of “hyperexcitability” include some pain syndromes as well as epilepsy. The former yields too much sensation, and the latter yields too much muscle contraction; the symptoms depend on which classes of neurons get hyperactive. Often, the best medications for such conditions promote the inactivation of sodium channels. Even people without pain syndromes may be familiar with the pain-relieving effect of blocking sodium channels by their experience with Novocain at the dentist’s office or lidocaine cream on sunburn. Medications for epilepsy are tailored not to shut down neural activity altogether but to constrain hyperactive neurons to accommodate.
6. Neurotransmitter receptors can shut down rapidly by desensitization, which is intrinsic to the protein, or by a short lifetime of the neurotransmitter itself, as it is destroyed by enzymes or soaked up by neighboring glial cells. Drugs and toxins that interfere with these processes and prolong the action of neurotransmitters can have dramatic effects on the nervous system. Benzodiazepines and other anxiolytic drugs extend the duration of ion flow through channels opened by the inhibitory neurotransmitter GABA. Nerve gas prolongs the action of acetylcholine, the neurotransmitter that makes muscles contract.
7. The detection of spicy food is not done by neurotransmitter receptors in the brain but by chemical receptors at the periphery that respond to capsaicin, the naturally occurring chemical that makes chili peppers hot and painful. In an interesting twist on drug tolerance, capsaicin can be used as an ointment to desensitize and internalize receptors and relieve pain associated with conditions like arthritis and neuropathy.
8. This process is referred to as homeostasis, and much work has been directed toward studying “homeostatic plasticity” in neural circuits—the process by which neurons restore a basic set point of activity, even as the strength of the inputs that stimulate them varies. Turrigiano, G. Homeostatic synaptic plasticity: Local and global mechanisms for stabilizing neuronal function. Cold Spring Harbor Perspectives in Biology 4, a005736 (2012).
9. In the case of habituation, neurotransmitter receptors don’t desensitize, but the neurons releasing chemical neurotransmitters run out of neurotransmitter. Kandel, E.R. & Schwartz, J.H. Molecular biology of learning: Modulation of transmitter release. Science 218, 433–443 (1982).
10. Barbano, M.F. & Cador, M. Opioids for hedonic experience and dopamine to get ready for it. Psychopharmacology 191, 497–506 (2007).
11. An interesting illustration of a brain’s ability to disregard the familiar comes from electric fish, which have an electric sense that lets them detect electric fields. These fish actively explore their environment by emitting a signal called an electric organ discharge (EOD)—the fish’s own stereotyped “call,” which produces an electric field around the fish. If an object is in the vicinity, the electric field is distorted—perhaps loosely analogous to feeling the distortion of your skin when you press on an object. It’s the deviation of the signal from the usual that indicates the possibility of something worth fleeing from or investigating. The fish’s own EOD signals nothing of potential significance. Accordingly, neurons that generate the EOD also send signals within the fish brain indicating that they have done so. The signal is exactly the opposite of the sensory input that the fish receives from its own undistorted EOD, effectively neutralizing the fish’s sensation of its own “call” when there is nothing to be detected. Bell, C., Bodznick, D., Montgomery, J. Bastian, J. The generation and subtraction of sensory expectations within cerebellum-like structures. Brain Behavior and Evolution 50, 17–31 (1997).
12. Gomot M. & Wicker, B. A challenging, unpredictable world for people with autism spectrum disorder. International Journal of Psychophysiology 83, 240–247 (2012).