Why should the future resemble the past? Well, for one thing, it always has. But that is itself an observation from the past. As the philosopher David Hume pointed out in the middle of the 18th century, we can’t use our experience in the past to argue that the future will resemble it, without descending into circular logic. What’s more, physicists remain unable to explain why certain fundamental constants of nature have the values that they do, or why those values should remain constant over time.
The question is a troubling one, especially for scientists. For one thing, the scientific method of hypothesis, test, and revision would falter if the fundamental nature of reality were constantly shifting. And scientists could no longer make predictions about the future or reconstructions of the past, or rely on past experiments with complete confidence. But science also has an ace up its sleeve: Unlike philosophy, it can try to measure whether the laws of nature and the constants that parameterize those laws are changing.
The fine-structure constant, α, is among the most ubiquitous and important of the fundamental constants of nature. It governs how strongly light and matter interact. If it were even slightly different from its present-day value of about 1/137, the universe would look very different indeed—and would almost certainly be inhospitable to life. While the laws of physics permit α to vary over time, few thought that it actually did. That is, until 1999, when scientists conducted an analysis of the light reaching us from very bright, very distant astrophysical objects called quasars.
This analysis took advantage of the fact that the atoms of every element preferentially absorb or emit certain colors of light in a manner that intimately depends on the value of α. These absorptions and emissions can be seen as bright or dark lines when light is broken into a spectrum, as when a prism splits white light into a rainbow of color. As light from quasars passed through gas clouds on its way to us, certain atoms in the gas clouds imprinted dark absorption lines on the light’s spectrum, which were then compared to the same atomic absorption lines as produced and measured in a laboratory.
To the researchers’ surprise, when they compared the spectra of that ancient light with spectra produced in the lab, they found a discrepancy: a slight mismatch in the absorption lines. This suggested that billions of years ago, when the absorption of the quasar light by a gas cloud took place, α was smaller than it is now by about one part in 100,000. In other words, α had slightly increased over the past several billion years.
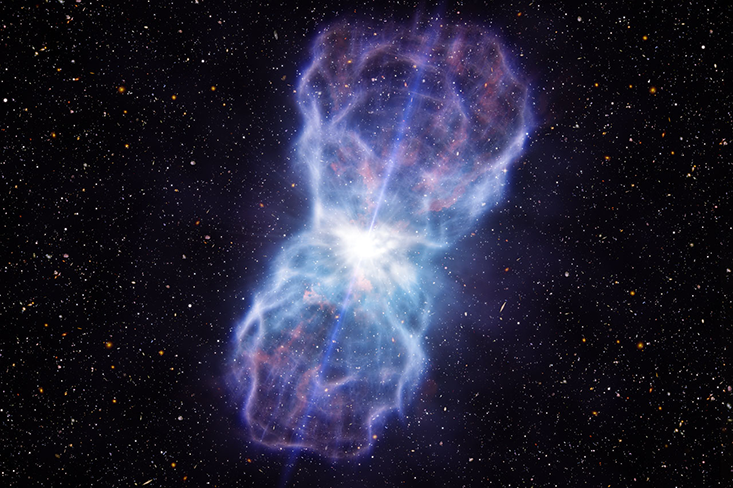
The possibility of a changing α was a bombshell that sent physicists scrambling for complementary approaches that could confirm or contradict the astronomical findings without relying on the same assumptions about the astrophysical environment. Fortunately for them, to observe how physics worked billions of years ago, you don’t have to look at ancient starlight from the heavens—you can also look at the very ground beneath your feet. Earth has been around for more than 4 billion years, and its ancient mineral deposits offer an alternative record of processes that took place billions of years ago. Variations in α would manifest themselves in fluctuating decay rates for various radioactive isotopes found in mineral deposits. The best measurements came from a 1.8-billion-year-old nuclear reactor that had been serendipitously discovered decades earlier.
In 1972, French nuclear scientists found something strange in samples of uranium ore from a mine in the Oklo region of Gabon, Africa. The ratio of uranium-235 (the isotope used in nuclear bombs and reactors) to uranium-238 (the more common uranium isotope) was smaller than what is typically found in geological deposits. This was quite puzzling, since, absent recent human activities, this ratio should depend only on how much uranium-235 was present in the primordial dust cloud from which Earth formed, and the time elapsed since then. Analyzing the Oklo rocks in more detail, scientists also found them full of a rich suite of radioactive products. The rocks had hosted a powerful, sustained, and ancient nuclear reaction.
How could nature have made a nuclear reactor in Earth’s crust? Man-made reactors rely on all sorts of containment and safety infrastructure to generate nuclear power, but, fundamentally, all one needs for a self-sustaining nuclear fission reaction is a large enough uranium sample that is at least 3 percent uranium-235. Then, just add water, which enhances the nuclear reaction by concentrating neutrons produced by the uranium’s radioactive decay. Today’s uranium-235 fraction is far too low for a natural nuclear reactor to spontaneously arise—but this wasn’t always the case. Uranium-235 decays faster than uranium-238, so its fraction increases as we go back in time. At 2 billion years in the past, it reaches a critical threshold that does allow a nuclear reaction to be sustained.
When they compared the spectra of that ancient light with spectra produced in the lab, they found a discrepancy: a slight mismatch in the absorption lines.
When it was running, the Oklo reactor produced large quantities of neutrons, some of which escaped and were captured by trace isotopes in the surrounding rock—a process extremely sensitive to the value of α, the fine-structure constant. By looking at the relative amounts of different isotopes found in the Oklo deposits today, scientists determined the value of α when the reactor was running. It was the same as today, within a certain margin of error. The heavens and the Earth seemed to be telling us conflicting stories about the distant past.
But both the astronomical and geological experiments were messy in their own way. Each depended on a host of assumptions about environments very far removed from us, either in space or in time. To achieve a cleaner measurement, researchers turned to an environment that they were able to control completely: their own laboratory. Atomic spectra have differing (and, in some cases, wildly differing) sensitivities to the value of α. By measuring these spectra over time, scientists could measure the time variation of α. The question is, how long would these measurements have to last in order to be useful?
The answer is not very long at all. Modern spectroscopy, the science of measuring absorption and emission spectra, is fantastically precise. It is so precise that a measurement over a few months or years would constrain the rate of change of α to a greater degree than either the Oklo or quasar measurements. These laboratory measurements found that, at least for today, α is not changing. The most sensitive of these measurements have determined that, if α is changing, it must be doing so by less than one part in 10 million billion per year. Extrapolated to a longer time period, that corresponds to a sensitivity of better than one part in a million over 10 billion years, which is about 10 times better than the sensitivity achieved by the 1999 astronomical observations of quasars.
The heavens and the Earth seemed to be telling us conflicting stories about the distant past.
So, is the case closed? Was the quasar data somehow flawed, and α proven to be fixed in time? Not quite. First of all, while the laboratory measurements rule out present-day changes in α that are on the scale suggested by the quasar data, they say nothing about the possibility of α changing in the distant past—the period of time for which the quasar data is relevant. Secondly, new astronomical data has complicated the picture in an unexpected way. An analysis of a newly measured set of quasar spectra, published in 2011 by the same group that authored the original 1999 paper, suggested that α in the distant past was slightly larger than the present-day value. This is in contrast to the previous astronomical observations of a smaller distant-past α described above.
Based only on these conflicting past values of α, one might be tempted to conclude that the astronomical results are simply unreliable, but the story is more subtle than that. The observations that yielded a smaller past α came from a telescope located in Hawaii, in the northern hemisphere, while the more recent observations that gave a larger past α came from a telescope in Chile, in the southern hemisphere, so the two telescopes have looked at widely separated directions in the sky. A combined analysis of all the data suggests that rather than a time variation of α, these observations are seeing a spatial variation—the value of α gets larger the deeper one looks in one particular direction, while growing smaller in the opposite direction. Because the deeper in space you look, the farther back you see in time, the spatial variation, if studied along only one direction, mimics a time variation.
The search for a changing fine structure constant reflects how extraordinarily difficult it is to consider and characterize a universe that may be changing in time at its most fundamental level. Because we always make measurements in the present, interpretations of data must necessarily make some assumptions as we try to unravel ourselves from the substrate on which we exist, and which we are also trying to measure. Whatever the ultimate truth about α turns out to be, its story is still being written.
Igor Teper is a physicist and an author.