Being an experimental scientist can sometimes seem like a thankless task. You may be used to reading headlines about experiments that end up making great discoveries, but less is heard about the (often heroic) efforts of experimentalists that have yet to detect or observe what they set out to.
Some of these efforts have spanned decades in time and generations of manpower and expertise. However, the absence of a result is often just as scientifically meaningful as any popularized discovery: We learn more about what the natural world isn’t, or doesn’t have. Getting a positive signal from any of these, though, would have far reaching consequences for our understanding of the universe, or our place in it.
Below is a list of seven ongoing experiments that have yet to find what they’re looking for. All of them are remarkable for their ingenuity and ambition. It’s no wonder where the perseverance to push on with these experiments comes from.
Nature seems to have a regular penchant for mocking scientists’ hopes and expectations.
To shine a light on dark matter, bury a tank of liquid xenon underground
Scientists theorize that filaments of dark matter form the scaffolding which all the galaxies we can see cling to. And surrounding each galaxy is a dark matter halo, which provides the extra gravity needed to account for how stars rotate around their galactic cores. Yet, dark matter is something we have yet to directly detect. Although the past decades have seen numerous attempts to detect dark matter through possibly very weak interactions with ordinary matter, all have come up empty.
Among the various forms dark matter could take, so-called Weakly Interacting Massive Particles (WIMPs) represent one of the more compelling possibilities to particle physicists. The LUX experiment, located almost a mile underground in a former mine in South Dakota, has, among others, helped set the bar high for failing to detect WIMPs. Encased in a tank filled with 72,000 tons of high-purity water to filter out unwanted cosmic rays, LUX consists of about a third of a ton of liquid xenon surrounded by detectors sensitive enough to detect the light emitted when dark matter collides with a single xenon atom.
LUX’s failure to detect any traces of dark matter has spurred the LUX-Zeplin upgrade—an experiment containing about 20 times the amount of liquid xenon as LUX. Whether the upgraded experiment finds any success where LUX didn’t remains to be seen. Nature seems to have a regular penchant for mocking scientists’ hopes and expectations.
To (really) see the Big Bang’s leftover gravity waves, look at different frequencies
Gravitational waves (the gravitational analogue of electromagnetic radiation, or light) from the time of the Big Bang would have left a unique trace in the cosmic microwave background, the relic radiation left over from the explosion that began the observable universe, which we can see in every direction. It shows tiny fluctuations in its temperature and polarization, which provides us with a snapshot of the gravitational field at the very epoch—when the universe was 379,000 years old—that neutral hydrogen first formed. This trace would have come in the form of a rotational pattern of polarization, technically known as “B-modes.”
Despite the excitement the BICEP/Keck collaboration generated by announcing, in 2014, the detection of such B-modes, their origin turned out to be something rather more mundane. What appeared to be primordial gravitational waves were really polarized dust particles at high galactic latitude, which can mimic the same rotational pattern of B-mode polarization as gravitational waves if present in large enough amounts.
Undeterred, the BICEP collaboration has upgraded to the BICEP3 configuration, which consists of an array of about 2,500 detectors (so-called bolometers) designed to observe the cosmic microwave background at a much lower frequency than its previous incarnation. A decade’s worth of observation over the various versions of the BICEP telescope still hasn’t garnered a positive detection of B-modes from primordial gravitational waves, but the search won’t stop any time soon—and the competition to detect it first is only just starting to heat up.
To see if the strong-nuclear and electroweak forces are united, look for light’s “sonic boom”
The standard model of particle physics is a culmination of decades of interplay between theory and experiment, from the inception of quantum mechanics to the proposal that the weak nuclear force (the force responsible for certain types of radioactive decay) and electromagnetism are different aspects of a single unified “electro-weak” force. The electromagnetic and weak nuclear forces only appear distinct to us at the scales of a typical laboratory experiment we’re familiar with because the Higgs field—which confers mass to particles that interact with it—hides the underlying symmetry governing these two forces.
Cherenkov radiation—the optical equivalent of sonic booms.
Within the standard model there is another force, the strong nuclear force, that we expect to be unified with the electroweak force at energies about a trillion times higher than what can be reached at CERN, in a process known as Grand Unification. One of the predictions of Grand Unified theories is that the proton ceases to be a stable particle, and can decay into other particles—such as pions and positrons—albeit incredibly rarely, with a half-life that can approach a hundred trillion trillion times the age of the universe in some models.
Super-Kamiokande—and its planned upgrade, Hyper-Kamiokande—located a kilometer under a mountain in the Kamioka laboratory in central Japan, have been charged with looking for (among other things) the tell-tale signatures of such extremely rare proton decays in gargantuan tanks of super pure water. By scanning for faint flashes of light known as Cherenkov radiation—the optical equivalent of sonic booms—Super-Kamiokande looks for the very energetic particles that the proton decays into.
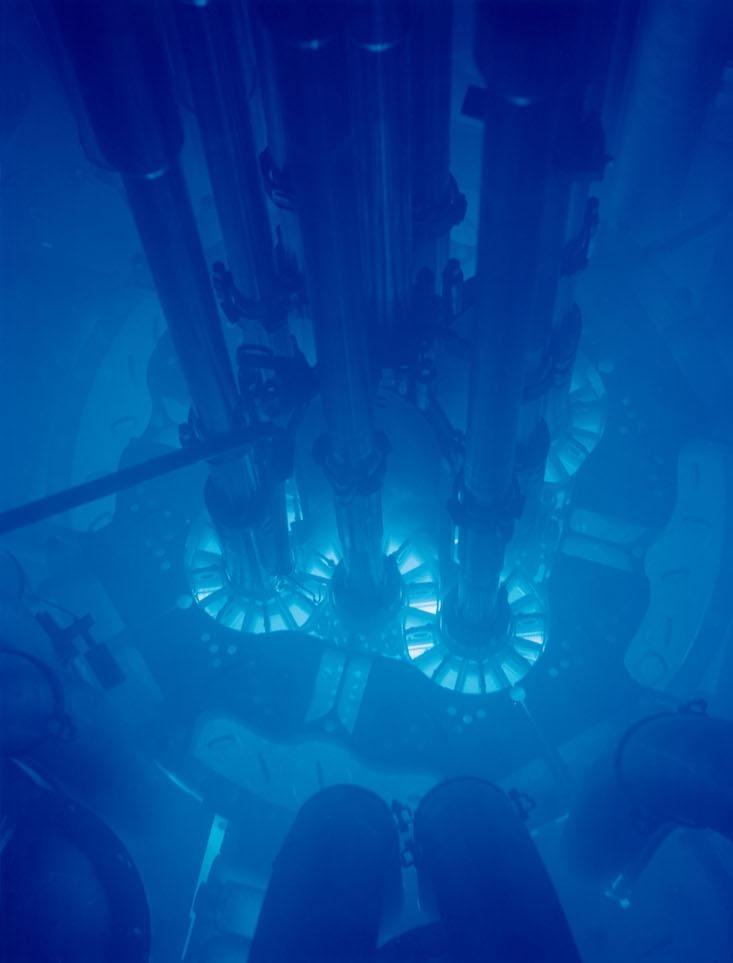
So far, nothing. But Hyper-Kamiokande, which is aiming for a 10-fold increase in sensitivity compared to its predecessor, should start observing by 2020.
To test for supersymmetry, probe the neutron
The standard model of particle physics predicts that the neutron—which, along with the proton, makes up all atomic nuclei—has an extremely minuscule electric dipole moment (EDM), the fixed distance separating two opposite charges. Its smallness is presumably the reason why it hasn’t been detected. But theories that extend the standard model with supersymmetry—a hypothesized equivalence between force and matter—generically predict EDMs that can be 100,000 times larger in magnitude than the standard model prediction.
By placing bounds on the magnitude of the neutron EDM, one can test whether supersymmetry is present in nature in ways that are more stringent than what one might accomplish in particle accelerator experiments. CryoEDM, at the Institute Laue-Langevin in Grenoble, France, is one such experiment. By observing how the spin of ultra-slow neutrons “precess”—that is, change the orientation of its rotational axis—differently in the presence of magnetic and electric fields, one can make precise measurements of the neutron EDM, if present, since this precession rate differs if the neutron has an EDM.
By the time CryoEDM reaches its full design sensitivity, it would be in a position to all but rule out the existence of supersymmetry. On the other hand, any observed EDM would be tantalizing evidence that supersymmetry is indeed realized in nature, as the value implied by the standard model alone is far too feeble to be detectable with current experimental sensitivity.
To spot extra dimensions, give gravity a close-up
Extra dimensions, if they exist, can modify the way gravity acts at very short distances. Not only do they imply deviations from the usual inverse square law of Newtonian gravity, they also imply the existence of new short-range forces of gravitational strength which would violate the so-called equivalence principle. The equivalence principle states that all matter—a cannon ball, an apple—falls identically in a given gravitational field. The reason extra dimensions come with all this extra baggage is because the fields that control the size of the extra dimensions themselves mimic the force of gravity, but only at very short distances and do so differently for different kinds of matter.
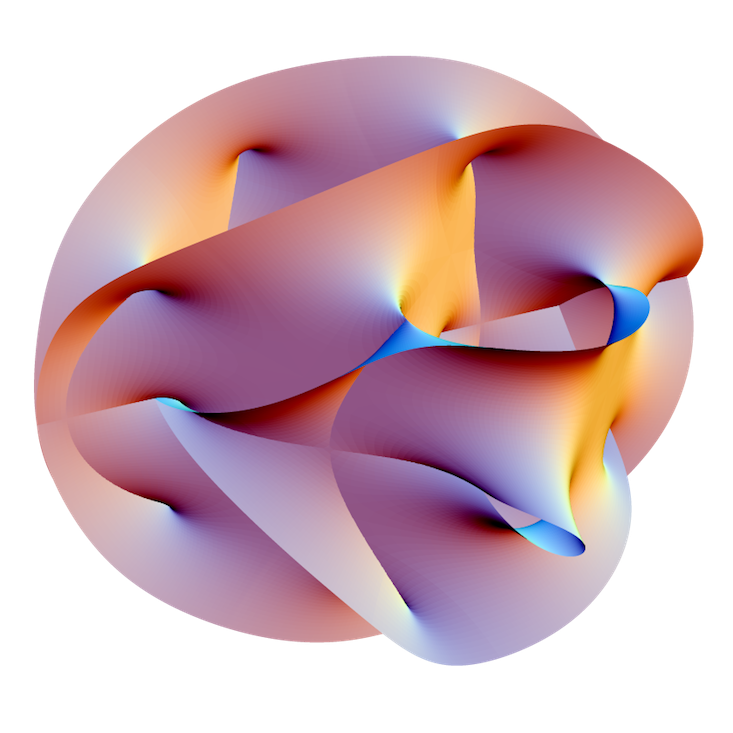
Although Einstein’s theory of general relativity has been thoroughly tested from solar system to cosmological scales, it wasn’t until recently that researchers began systematically testing it on sub-millimeter scales.
Using precisely calibrated torsion balances, the Eöt-Wash collaboration, (named in honor of Baron Eötvos, who pioneered such experiments at the turn of the 20th century) based at the University of Washington, have been searching for violations of the equivalence principle—in addition to deviations from the inverse square law of Newtonian gravity—at scales that are now approaching a few 100,000ths of a meter. So far, no modifications to Newton’s laws, nor the equivalence principle, have been found, suggesting that extra dimensions, if they exist, are curled up into regions far smaller than a few tens of a micron.
To glimpse the cosmological “dark ages,” tune in to a faint radio signal
There is an epoch in the history of the universe that we know relatively little about—the so called dark ages. This is the era after “recombination,” when neutral hydrogen first formed and before the first stars started to shine.
A hydrogen atom sitting by itself doesn’t emit very much. However, like any planet orbiting a sun, which also rotates around its own axis, the solitary electron that orbits any given hydrogen nucleus “spins” on its own axis either aligned with, or against, its orbital motion. The latter has less energy.
Backlit by the cosmic microwave background, the neutral hydrogen in the dark ages has a certain fraction that have been excited to spin in the higher energy aligned configuration. As these excited atoms transition to the lower energy anti-aligned configuration, they emit radiation at about 1.4 gigahertz, corresponding to a very faint radio signal with a wavelength of about 21 centimeters. Detecting this so-called 21 centimeter background would allow us to peer directly back into the dark ages.
The Low Frequency Array (LOFAR) telescope is an array of about 20,000 phased antennae located throughout Europe (though mainly in the Netherlands) that has been peering out into the cosmos since 2012 in the hopes of detecting this very faint signal. However the earth, and the galaxy that it sits in, is a very noisy place, and it has so far been impossible to detect any signal from the dark ages that rises above the local noise. Ambitious plans are afoot for an international array known as the Square Kilometer Array (SKA) to go further, but for now, the dark ages remain just that.
“Two possibilities exist: either we are alone in the universe, or we are not. Both are equally terrifying.”
To discover aliens, just never stop listening
Conclusive proof of the presence of intelligent life in the universe other than our own would be a watershed moment for civilization. A collective effort, involving a range of experiments, has been searching for signals of extra-terrestrial intelligence for nearly as long as radio technology has been around. The underlying idea is that artificial radio signals can be distinguished from those created by natural (astrophysical) sources because of their narrow range in frequencies and repetitive nature, as is the case with human radio transmissions. A tantalizing candidate signal appeared in 1977, although it has never been seen again and a natural explanation can’t be ruled out.
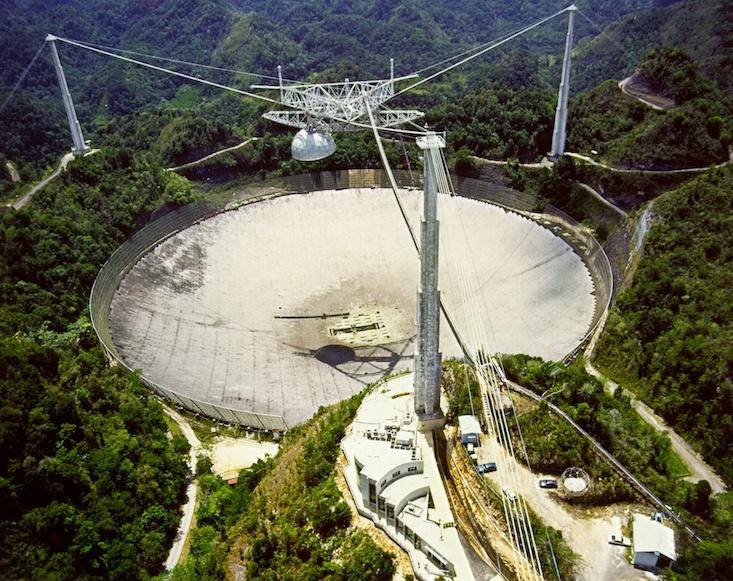
SETI, the Search for Extra-Terrestrial Intelligence, continues with a variety of dedicated radio telescopes, including the Allen Telescope Array, which has recently been supplemented with technology nominally deployed for the search for exoplanets. Scientists have also purposed it to look for alien mega-structures, hypothesized by the physicist Freeman Dyson, that advanced, civilizations might use to harvest energy directly from their host stars. Despite decades of coming up empty, the collective search for extra-terrestrial intelligence is now better equipped than ever to adress Arthur C. Clarke’s famous anxiety that “two possibilities exist: either we are alone in the universe, or we are not. Both are equally terrifying.”
Subodh Patil is a theoretical physicist at the Niels Bohr Institute at the University of Copenhagen. He tweets on occasion at @_subodhpatil.
WATCH: The theoretical cosmologist Laura Mersini-Houghton on whether her work is the first empirical test of string theory.
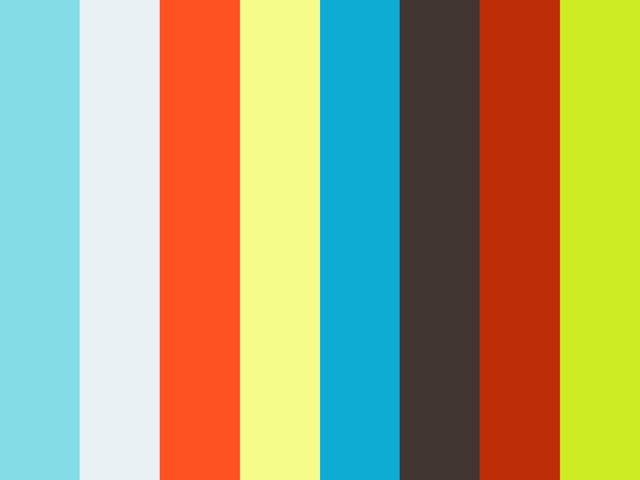