It was mid-May of last year when we realized we were onto something.
We were measuring the electrical properties of a pellet squeezed between two diamonds in an anvil. The anvil could apply 2 million atmospheres of pressure, or about half the pressure found at the center of the Earth. Theoreticians had calculated that at extreme pressures like these, and temperatures between 123 degrees Celsius and negative 73 degrees Celsius, metallic hydrogen would lose all of its resistance to electricity. It would become a perfect conductor, letting current flow through it essentially forever.
This could be a big deal. True room-temperature superconductivity could allow lossless electrical transmission, fast trains, levitation, new computers—the sky would be the limit. But superconductivity at room temperature was (and remains) unreachable—when we were doing our experiments, nothing warmer than negative 130 C worked at normal pressures.
So, inspired by the theoretical prediction, we set about looking for a chemical compound that had a lot of hydrogen in it. We tried silane (four hydrogen atoms bonded to one silicon atom), but it became superconducting at an extremely cold negative 256 C. Not impressive.
Nobody imagined that people would find superconductivity in cuprate systems.
We eventually turned to hydrogen sulfide, which consists of hydrogen atoms squeezed into a lattice of sulfur atoms. Theorists predicted that it would become superconducting at negative 193 C—also not that impressive. And it was toxic and smelly, so smelly that it’s added to natural gas to warn people of leaks.
But hydrogen sulfide was readily available. So we made a tiny pellet of it, about the width of a human hair. On May 17 we clearly saw a transition to superconducting behavior, or zero resistivity, at a temperature of negative 223 C. It was colder than the prediction, and far colder than the best superconductors.
But the result encouraged us and we set about trying to reproduce it. Over the next three months of very hard work, we hovered at the frigid temperature of minus 220 C, studying the details of our superconducting system, playing with pressure and temperature.
Then we had some lucky breaks.
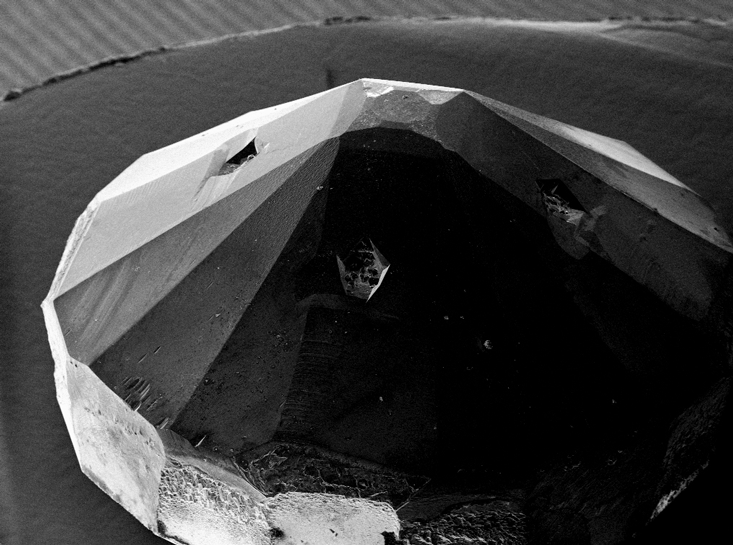
The history of superconductivity is full of near-misses, luck, and unexpected discoveries. Kamerlingh Onnes, who won the Nobel Prize for being the first to see superconductivity, wasn’t actually looking for it. He had set out to prove that the resistance of metals gradually approach zero as they cool, and become zero at absolute zero temperature. Instead, he saw the resistance of solid mercury suddenly decrease to nothing at 4 degrees above absolute zero. What’s more, Onnes was also the first to see helium transition into a superfluid, which can flow without resistance. But he missed the superfluidity completely, because he wasn’t looking for it. It was later discovered by Pyotr Kapitsa in 1937, winning him the Nobel Prize. Kamerlingh’s original experiment had two Nobel-worthy results in it!
The discovery of superconductivity in cuprates (which are ceramics doped with metal atoms) was also entirely unexpected. Most of the scientific community spent many years trying to increase the superconducting transition temperature of metal alloys. Nobody imagined that people would find superconductivity in cuprate systems—until, that is, they became world-beaters, and took the record for high-temperature superconductivity with temperatures around negative 135 C.
Thankfully, we had our own dose of luck, in a couple of different ways. On August 22, the pressure loading mechanism in one of our diamond cells prevented us from increasing pressures at low temperature. So we began to warm up our sample. As we watched the data come in, we saw something entirely unexpected: The sample’s resistance was decreasing with warming! The temperature at which it was transitioning to superconductivity was much higher than we thought it was. We were surprised to see the transition reach negative 120 C.
The result forced us to look again at the data from our earlier experiments. When we did, we found very weak signs of superconducting transitions as high as negative 100 C —a world record. These earlier measurements were not done under ideal circumstances, using three electrical contacts instead of the four that we’d need to be secure in the data. But even that early, rough data suggested there was something entirely new happening in our sample.
We would later learn that our hydrogen sulfide (H2S) pellet was unexpectedly dissociating into sulfonium (H3S). This process was completely missed by theoretical calculations, and we didn’t expect it. But this dissociation was critical. The hydrogen atom in sulfonium is squeezed by the surrounding sulfur atoms in an effect called chemical compression, effectively increasing the pressure we were applying. At the same time, sulfonium can still essentially be considered to be plain metallic atomic hydrogen, so that we could compare it to theoretical predictions for that material. About 92 percent of its superconducting behavior is determined by the lattice of its hydrogen atoms alone, and only 8 percent comes from the sulfur atoms holding the hydrogen atoms in place.
As we continued to chip away at our experiment, we saw warmer and warmer temperatures. The warmest superconducting transition observed to date had been negative 110 C, for a cuprate under pressure. Our approach allowed us to reach a transition of negative 70 C.
Now, that is still a very cold temperature. But keep in mind that the field started at a temperature of negative 269 C. Temperatures of negative 70 C are dramatically higher. In fact, they actually occur outdoors in various places on Earth, like in the winter in Yakutsk, Russia, where a temperature of negative 64 C was once recorded. For some people, this is already room-temperature superconductivity—or to be more precise, street-temperature superconductivity. With any luck, we’ll move to a more southerly city soon.
Mikhail Eremets and Alex Drozdov are physicists at the Max Planck Institute for Chemistry in Germany.