The Fermi paradox, the “where is everybody?” puzzle, is a persistent question in the search for life in the universe. It asks why, if life is not exceedingly rare in the cosmos, it hasn’t shown up on our doorstep. Equally we might ask why we haven’t even heard from alien life, through radio signals or any other means. A part of the answer could be that our present work on the search for extraterrestrial intelligence is actually very limited. Estimates show that we’ve only examined the equivalent of a hot tub of water compared to all the world’s oceans in our combing through the electromagnetic information that rolls in from the cosmos.1
If you’re a glass-half-full kind of person you’ll see this as an opportunity, but the problem is that we don’t actually know what might be filling the glass in the first place. The vast majority of SETI studies look for structure in electromagnetic radiation, whether in amplitude or frequency modulations of radio waves, or regularity in pulses of light, or in multi-wavelength correlations. In other words, we assume that information might be sailing past us in representations built using classical physics. But what if that’s just wrong?
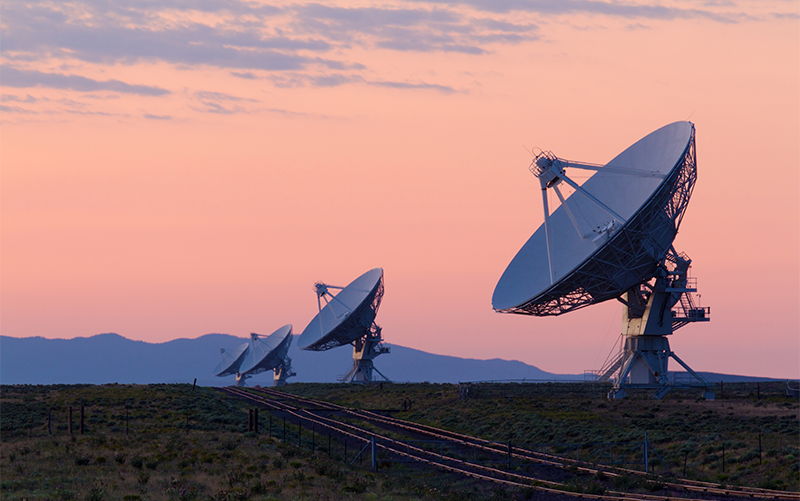
In recent years a small cadre of physicists and astrophysicists have examined the possibilities for communication across the universe that uses the quantum properties of matter and radiation.2 Here on Earth quantum mechanics is perhaps the greatest triumph and the greatest headache of 20th-century physics. As theories go it has repeatedly validated itself through some of the most exquisite measurements we’ve ever made about the world, yet it remains profoundly challenging because of its counterintuitive rules and contentious interpretations. Even the “simple stuff” is hard, including the basic mathematical tools needed to describe how matter and radiation futz around in weird states of uncertain superposition (think Schrödinger’s cat) or mind-bending entanglement, where properties are linked across space and time, yet never definite until interactions occur.
Nonetheless, these tools point to a rich array of possibilities for the transport of information and for computation, all based on intrinsic properties of the universe rather than the parochial approaches we have for things like digital bits and semiconductor physics. Sitting at the center of this is a mechanism that we call quantum teleportation, where a quantum state can be transferred between otherwise indistinguishable objects, so that it is exactly as if a material system has been relocated. That quantum state might be of a photon, or a particle, or many of these objects, and might involve properties such as particle spin or photon polarization. Quantum teleportation is very real and was first accomplished in 1997, becoming an almost standardized technique here on Earth.3 It has even been performed between a lab on the ground and an orbiting satellite, by teleporting the quantum state of single photons.4
To understand just what this could mean for cosmic communications, we have to wade a little deeper into the details, or else risk using a lot of metaphors and mystical sounding hand-waving. Rather than apologize for this I’ll say that it’s absolutely necessary to begin to grasp the far-reaching implications of quantum information exchange for thinking species.
The first step is to back up a distance and mention the “qubit,” or quantum bit. In classical information the “bit” is literally an object that can represent only two conditions, a 1 or a 0—like the way that the cluster of pixels or ink dots in front of you has just done. But the photons and particles of the universe are fuzzy, quantum things with both wavelike and particle-like behaviors and can exist as indefinite superpositions of 1s and 0s, or a mix of spins or polarizations and other qualities. These are qubits, and Schrödinger’s famous cat, that is both alive and dead simultaneously in a state of superposition, is very much a qubit. Qubits can be manipulated in ways that maintain their fuzzy superposition but nonetheless modify their unknown quantum state (like rotating a cat’s box without opening it). These manipulations are cousins to the kind of logical gate operations we perform on classical bits of data with things like AND, NOT, and OR gates buried in our silicon chips, so that we’ve come to term them as quantum gates, forming the basis for quantum computation using qubits.
Qubits can be connected using another mind-bending property of the world that we call quantum entanglement, where (for example) a pair of qubits can be intermingled so that their unknown properties are nonetheless knotted together, and when one qubit is finally measured the consequences of that measurement will be instantly propagated across its entangled partners, no matter where they are. For computing this is key, because the application of quantum gates will naturally propagate across all entangled qubits.
If you’re a glass-half-full kind of person, you’ll see this as an opportunity.
If you’re keeping up so far that’s good, because we’re almost ready to see how all of this comes together in quantum teleportation and interstellar communications. The last piece to mention is called the “no-cloning theorem,” which is not some edict from the Star Wars mythology, but rather a mathematical proof that an unknown quantum state cannot have an identical, independent copy made of it or else the limits of the speed of light for communication will be broken, with all hell breaking loose for causality and reality as we understand it. To get around this, quantum teleportation must destroy the original unknown quantum state as it is copied.
Now imagine that we have Bob, sitting here on Earth, and Alienx, sitting on a planet around a distant star. Alienx wants to teleport some quantum information—the unknown state of a single qubit that we’ll call Q—to Bob. Alienx generates an additional, entangled pair of qubits and sends one of those out across space to Bob. In the meantime, Alienx applies a quantum gate to their half of the entangled qubit, but that quantum gate is controlled by the qubit Q that they want to send. In other words, they’re imprinting qualities of Q onto the entangled qubit (actually further entangling it). They then make a measurement of Q (necessarily destroying its quantum state in the process) and the manipulated qubit, storing the results in some classical bits and transmitting that classical data to Bob by any ordinary means, like a radio signal.
What Bob ends up receiving is one of the entangled qubits, and the classical data about Alienx’s measurements. He then reads the classical data and depending on what it says he applies a quantum gate to the received qubit, which will then acquire the state of the original Q. Lo and behold, an unknown quantum state, and quantum information, has been “teleported” across space. If that doesn’t sound exciting, consider a more elaborate example where the quantum states of a collection of atoms are teleported using the same principles. If those atoms were parts of a set of molecules, Alienx could transmit not just a boring chemical formula or molecular structural configurations, but the fuzzy quantum state of the molecules that they are utilizing, with precisely the same propensities for reaction and interaction. That is something completely out of reach for any classical communication.
The rub, though, and there is one, is that for any of this to work the pair of entangled qubits must retain coherence. Quantum coherence can be thought of as a system holding on to its pure quantum properties. For example, in the famous double-slit experiment an electron can exhibit pure wavelike characteristics of interference that modulate where the electron (as a particle) appears after passing through two tiny slits in a screen, and that wavelike interference only occurs when things are coherent, or undisturbed. Nudge the electron on its journey, or let it interact with the world in any other way and it can decohere, removing or collapsing its wavelike qualities to behave like a boring classical object.
The biggest barriers to coherence across the cosmos come from the fact that space contains photons and subatomic particles, as well as interstellar atoms and dust. Sending delicate, coherent quantum systems through all of this tends to make them far less coherent, causing the loss of their purest arrangements of superposition or entanglement—the intermingling of states that enables teleportation and communication. In a recent paper published in Physical Review D in April 2022, two scientists, Arjun Berera and Jaime Calderón-Figueroa of the University of Edinburgh have studied the effects of gravitational fields, interstellar matter, and cosmic radiation on coherence.5 What they find is that coherence could actually remain across interstellar distances, and perhaps even across the Milky Way galaxy for certain wavelengths of electromagnetic radiation. X-ray light is particularly promising for dodging many of the sources of decoherence across space.
Lo and behold, an unknown quantum state has been “teleported” across space.
But if aliens are sending out quantum hailing signals, how will we find them? Teleportation involves those two channels of communication, one classic and one quantum, and a signal would have to be repeated to stand any chance of reception by an unknown party. The quantum piece might be a series of single photons (the qubits) or clusters of photons in more complex, entangled arrangements, while the classical channel would consist of the repeating data on the measurements of the qubits that our Alienx made in the example used above. If these two kinds of electromagnetic signals arrive at the same time, and from the same place in our skies, we—or any other species—might realize we’re getting pinged with a teleportation.
Of course, the next steps; to figure out the protocols of what quantum gates to apply, and even how to respond, may seem hair-raisingly uncertain and complicated. Yet in truth, this would not be any more or less challenging than deciphering a classical extraterrestrial communications channel, and in some ways could be easier initially because we’d know that we were decoding a quantum communication and would have an idea of what possible quantum gates to try, from simple to more complex. In that sense we can already congratulate ourselves, because the use of quantum channels for communication could represent a filter between more sophisticated species and the classical plebeians of the cosmos, who are perhaps not worth investing time in.
But the real eye-opener is the implication that the best kind of transmitters and receivers for quantum teleportation communications would actually form parts of quantum computing systems. The process of qubit entanglement and the application of quantum gates for teleportation are simply elements of quantum computation. That tells us that, in principle, interstellar quantum communications could involve vastly richer information content than anything possible classically.
It’s possible, then, that this is the solution to the Fermi Paradox.
In fact, if issues of decoherence can be overcome, there is no reason why a quantum computer could not be distributed across interstellar space, with its entangled pieces interacting in much the same way as distinct pieces of a physically co-located quantum computer interact. In some respects, interstellar space is a much less decohering environment than pretty much anywhere on a planet like Earth, since the density of matter is typically around a trillion, trillion times lower (of course this is somewhat offset by the huge distances that quantum states have to traverse).
The transmission of computing information is still limited by the speed of light, so this kind of interstellar computing would be collectively slow. But that might not matter if the overall value of the process is vastly amplified by its distribution and the consequent access to greater computational resources (just more qubits for example). There might be forms of information exchange or problem-solving that are very much worth it, especially if they relate to deeper interspecies understanding or vital survival knowledge, which could include finding solutions to otherwise intractable scientific questions.
It is therefore possible that the solution to Fermi’s paradox, at least for interstellar communications, is that the language being used by anyone worth talking to utilizes quantum physics, does not look much at all like our parochial classical electromagnetic signaling, and has thus far evaded our scrutiny. A corollary to this would be that any communicating species might settle down to deeply encrypted, eavesdropper-proof computing exchanges that last for ages and are wholly inaccessible to the rest of us, even while representing the greatest flow of information in the universe. It could be worth our while to try to find out whether or not this is going on, and even if it isn’t we might learn something useful about our own baby-steps in utilizing quantum information.
Caleb Scharf is an astrophysicist, the director of astrobiology at Columbia University in New York. His most recent book is The Ascent of Information.
Lead image: Metamorworks / Shutterstock
References
1. Wright, J.T., Kanodia, S., & Lubar, E.G. How much SETI has been done? Finding needles in the n-dimensional cosmic haystack. arXiv 1809:07252 (2020).
2. Hippke, M. Searching for interstellar quantum communications. arXiv 2104:06446 (2021).
3. Science news staff. Behold the quantum teleporter. Science (1997).
4. Ren, J.-G., et al. Ground-to-satellite quantum communication. Nature 549, 70-73 (2017).
5. Berera, A. & Calderón-Figueroa, J. Viability of quantum communication across interstellar distances. arXiv 2205:11816 (2022).