In the 1958 science-fiction movie The Blob, an amorphous alien hitchhiked a ride to Earth on a meteorite. Upon landing, the translucent extraterrestrial began to devour humans, growing larger and redder with each meal. While the amoeba-like alien in the cult classic was famous for its insatiable hunger, The Blob managed to articulate something that previous science-fiction movies hadn’t: There’s no guarantee aliens will look anything like the lifeforms that we are familiar with.
Scientists and philosophers have been attempting to define life for ages. In biology class we were taught to define life through the set of features that we, and every other species on the planet share. Things like movement, respiration, growth, and reproduction. Life is made of cells and has DNA. But does biochemistry constitute the whole picture? As far back as 1970, Carl Sagan didn’t think so. Attempts at defining life, he and many others thought, were too constrained by the characteristics of life as we know it. A single example of extraterrestrial life could change everything.
Employing an elegant music metaphor, Sagan wrote, “It is not known whether there is a vast array of biological themes and counterpoints in the universe; whether there are places that have fugues, compared with which our one tune is a bit thin and reedy. Or it may be that our tune is the only tune around. Accordingly, the prospects for life on other planets must be considered in any general discussion of life.”
“Stars themselves could meet the criteria to be considered life.”
Today a new generation of astrobiologists have taken Sagan’s prospect to heart. If life has managed to gain a foothold somewhere else in the cosmos, alien life could look very different than the varieties of life found on Earth. If we don’t have a broad, robust definition of what life is, we may miss it when searching for extraterrestrial life in the cosmos.
In a white paper submitted to the Planetary Science and Astrobiology Decadal Survey 2023-2032, of the National Academies, a group of scientists, affiliated with NASA, SETI, and universities around the world, outlined a way forward in astrobiological research. They wrote “the probability that life in the universe would share a biochemical ancestry with life on Earth quickly diminishes the further away from Earth we explore.” It’s imperative, the scientists continued, “that we build foundational knowledge for life detection strategies that target universal biosignatures.”
Aaron Goldman, a biologist from Oberlin College, who has been working on problems relating to the origin of life on Earth, says it’s important we distinguish what we mean when we talk about living and non-living entities, especially in the context of astrobiology. “The most successful definitions of life fall into two general categories: entropic definitions, which describe life through its ability to increase internal order at the expense of increasing disorder in the surrounding environment; and evolutionary definitions, which describe life through its ability to evolve by natural selection,” Goldman says.
Entropic definitions detail life’s ability to harness sources of free energy, such as the sun, that feed metabolic processes which allow life to build structures and carry out its functions. The set of objects we call “life” are highly ordered and are low in entropy, according to this view.
But the entropic view of life is not without its problems. A counterintuitive result of thinking about life this way means stars themselves could meet the criteria to be considered life. Nuclear fusion and fission in the star’s core maintain the structures and processes that are necessary to its stable physiology and anatomy—a type of stellar metabolism that mirrors the metabolic processes of biological organisms. It seems obvious, though, that stars are not living, or at least they are not in the same class of entities that we might call life. Similarly, it’s easy to see the appeal of evolutionary definitions of life as a self-sustaining system capable of Darwinian evolution. Evolution by natural selection has driven diversity and adaptation in all living things on Earth since the last universal common ancestor.
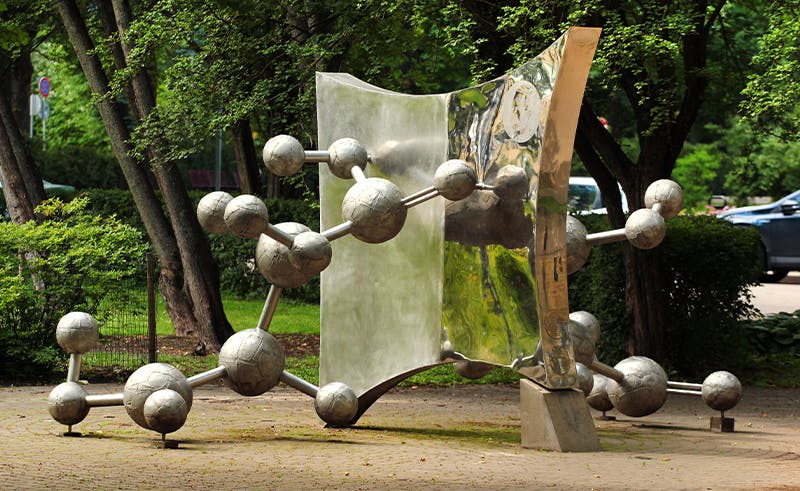
The trouble with qualitative descriptions of life, like the entropic and evolutionary approaches, is they often present numerous gray areas and strange counter examples. In such cases we are left with a set of life’s features that serve as a litmus test for whether one set of phenomena qualifies as life or not. Are viruses alive? Being unable to replicate without infecting a host, and their lack of energy needs, might suggest they aren’t under both the entropic and evolutionary views. What about digital or synthetic lifeforms? A lot of people might say no because they don’t share the biological features with the entities that we do call living, and are composed of an entirely different substrate. Under one such set of criteria, our answers might say no, and under another they might say yes, while our intuition might tell us something else altogether.
In recognizing the problems that can crop up when trying to apply these universal qualitative definitions of life, astrobiologists have asked, Are there quantifiable features of chemistry that can distinguish the characteristics of life in a way that can be applied in our search for extraterrestrial life? Could it be possible to build a “physics of life” from the ground up?
One of the first attempts at setting out a quantifiable framework for identifying extraterrestrial biological processes was made in 2004 by NASA planetary scientist Chris McKay. McKay introduced the “lego principle,” which describes the blocks of molecules that constitute structures in biology, such as proteins. McKay noticed that in contrast to abiotic processes, biology does not draw from the full range of available organic molecules when producing structures associated with life. Amino acids, which are probably the most important set of organic molecules used in Earth-based living systems, have the quality of being chiral. This means they have a non-superimposable mirror image, or that each amino acid has a left-handed and a right-handed version of itself. Of the 20 amino acids used in proteins, Earth-based life only makes use of the left-handed version, whereas abiotic processes tend to use an even distribution of left-handed and right-handed organic molecules.
McKay’s lego principle could be applied in the search for alien biochemistry in the solar system. Organic material sampled from promising sites for life, like Mars or Europa, could be tested for the chirality of one type of organic molecule over the other. Analyzing the concentration of organic molecules in these environments may reveal patterns indicative of life, even if those patterns do not involve the specific organic molecules exploited by Earth-based life.
Astrobiologist Sara Walker from Arizona State University and chemist Lee Cronin from the University of Glasgow have their own view of life. Their “assembly theory” suggests there is a quantifiable difference in the complexity of molecules that can be created by living processes compared to non-living ones. “Basically the idea is what underlies life is the physics that builds complexity in the universe,” Walker says.
Could it be possible to build a “physics of life” from the ground up?
In a rudimentary way, for an atom to form a bond to create a molecule, an energy barrier has to be crossed, so as a molecule gets more complex, it gets increasingly unlikely that it was created by chance; or as Walker puts it, “the universe doesn’t make complex things for free.” Life, though, is able to bypass these energy barriers, opening up an extremely vast chemical space where a mind-boggling number of complex molecular structures can be realized. The goal of assembly theory, says Walker, is to “understand the circumstances when selection emerges and when you start building up complexity.”
Assembly theory describes complex molecules with something called the molecular assembly index. The molecular assembly value of a molecule is determined by the shortest number of steps that are needed to create that molecule through its elementary building blocks (atoms and their bonds), and this number can be derived for a given molecule through analysis in mass spectrometers. Finding collections of molecules in space above a certain MA threshold would highly suggest the presence of processes that we might call living. For assembly theory, it is the intrinsic complexity of an object which determines whether it was the product of living processes.
“The perspective I have is that what we really need to do is not start from definitions of life and use those as guiding principles, but to try to start from thinking about the origin of life and what it means when life arises in a system,” Walker says. “What is fundamentally different when we talk about the general category of life and non-living things in the universe, and what sort of laws or principles would allow us to talk about that whole category and when that transition happens?”
Imagine human astronauts are scouring the surface of distant planets for signs of life. They come across an object that is undoubtedly a piece of alien technology. As a highly ordered and complex structure, we could not assume that it had randomly appeared into existence by chance. To properly explain its existence, we would have to tell a multibillion-year-long story, explaining how geochemical cycles on the planet evolved into life, which then evolved into intelligent life that eventually developed technology which was capable of building the alien hardware. “Cell phones don’t randomly fluctuate out of a vacuum,” quips Walker.
This example illustrates that the more complex a structure becomes, whether it is a piece of elaborate technology or even a molecule, the less likely it is that structure appeared by chance. Instead, a process which can use information to build complex structures has to be responsible for the creation of such complexity, and Walker and Cronin call that process life. Human technology and any potential alien technology is an extension of living processes and are part of the same lineage of complex objects.
The difference between what assembly theory calls living processes and non-living processes is that living processes are governed by information conserved through a history of interactions between an entity and its environment, whereas non-living processes are largely determined by chance. The mechanism for recording the history of interactions in the living entities we are familiar with is DNA, but that mechanism could look different in other varieties of life.
“The universe doesn’t make complex things for free.”
The main hurdle for applying assembly theory in trying to identify extraterrestrial life is that, at least currently, measurements must be made in situ, or on site. While NASA has plans to send mass spectrometers to Titan and Europa in the hope of identifying living chemistry, Walker thinks they will not have the high-resolution capacity to make the measurements that assembly theory would require. We are limited with how far we can send our instruments now, and there’s a big universe out there with lots of possible locations for life. Walker notes, however, that researchers working on assembly theory are developing ways the framework can be applied in the spectrographic analysis of exoplanet atmospheres.
One of the most talked-about methods that scientists will use for life detection is to look for atmospheric biosignatures, or chemical byproducts of living processes in the atmospheres of alien worlds. Life on Earth is responsible for a lot of what makes up our atmosphere, and by analyzing the atmospheres of planets outside our solar system, scientists hope we might detect signs that indicate the presence of life on those planets.
In 2020, scientists reported on the detection of phosphine (a possible biosignature) in the atmosphere of Venus. Did the Venutian clouds host life? Further measurements were unable to detect the gas, while another study suggested the initial detection may have mistook phosphine for sulfur dioxide, a common gas that isn’t a sign of life. A 2021 study highlighted the possibility that abiotic volcanic processes could explain the amounts of phosphine astronomers initially observed.
The uncertainty around the discovery of phosphine, and its possible abiotic sources on Venus, underscores some of the problems with the atmospheric biosignature method of life-detection. What’s more, it leans on our understanding of Earth’s biochemistry to form assumptions about how alien lifeforms may affect their home world. An interesting way of getting around this was presented in a 2022 paper by philosopher David Kinney and SETI principal investigator Chris Kempes. They suggest we should look at planets with the strangest atmospheres.
Kinney and Kempes think we should collect as much atmospheric data from as many exoplanets as possible to create a profile of the chemical composition of those planets. From there, our life detection efforts should be focused on worlds that are statistical outliers, or anomalous. Kinney and Kempes’ approach isn’t hung up on defining life in a particular way, but central to their argument are the core assumptions that living organisms affect the atmospheric chemistry of their planets, that life in the set of observable exoplanets is rare, and that there aren’t common abiotic processes that mimic biological ones.
So far, of course, we don’t have smoking-gun evidence of extraterrestrial life. But Sagan would be pleased that today’s astrobiologists have expanded the perspectives on what life might be. Like the transition in our understanding of gravity from Newton to Einstein, a more powerful explanation of what life is as a system, and the conditions from which it could emerge, will better prepare us to answer the question, Are we alone in the universe?
Conor Feehly is a freelance science writer based in Auckland, New Zealand. His writing has been featured in New Scientist, Cosmos Magazine, Discover Magazine, and others.
Lead image: Tithi Luadthong / Shutterstock