When I began studying how animals swim, I didn’t feel much like a physicist. I’d just finished my bachelor’s in physics during which time I’d been taught that physicists work on one of a handful of buzzwords: quantum mechanics, cosmology, gauge theory, and so on. To see if graduate school was right for me, I shadowed a friendly research group at the University of California, San Diego—but they didn’t study any of these buzzwords. They used high-powered mathematics to understand things like the locomotion of snails, worms, and microorganisms.
I was grateful for the opportunity, and I thought the problems they studied were beautiful and interesting—just not fundamental physics. As I became more involved in the group, this distinction grew into an identity crisis. Theoretical physicists are kind of like artists, or athletes: If you feel yourself drifting further from Klee or Peyton Manning, it can seem like a catastrophe. I thought I could feel Einstein and Feynman looking down at me and frowning as I took a turn down the wrong path.
It would take some impressive feats by microorganisms to convince me that they were as sexy as smashing atoms together—and they did not fail to deliver. Some are capable of shooting small needles, or even segments of their DNA, which accelerate about 1,000 times faster than a space shuttle launch; others share genetic information with their unrelated neighbors, forming an Internet many millennia older than ours; many outlive us, despite being 1 million times smaller. Even more interesting was that microorganisms do not obey Newton’s laws, which govern basic motion and are a pillar of classical physics.
These remarkable facts changed not only how I perceived bacteria, but how I defined what it meant to be a physicist.
Microorganisms experience their fluid environments in a radically different way than we do. We experience water as we do only because we are a certain size, and swim at a characteristic speed. Physicists and engineers describe how water might “feel” with the Reynolds number, which compares the relative importance of inertia and viscosity (drag) for a given liquid flowing at a given speed. The larger this number is, the more important inertia is relative to drag. Turbulence (the white, choppy, bubbly water you see around speedboats) kicks in when the Reynolds number is big, at least a few hundred.
By this you can infer that human swimming is characterized by a number that is somewhere less than a few thousand for wimpy swimmers like me, and somewhere more than that for Olympians. For bacteria, the Reynolds number turns out to be roughly 0.001. That means inertia is almost 1 billion times less important to them than for us humans.1
To recreate the experience of a bacterium in water, a human of average size would need to be immersed in molasses and swim at about 1 centimeter per minute. If they suddenly stopped, they would coast less than a millimeter before coming to a halt; that is, the coasting distance would have to be measured with a microscope. The time spent coasting would also be miniscule, somewhere on the order of microseconds, which is the time between flashes in a strobe light. Similarly, a bacterium that was traveling at top speed and suddenly stopped would coast one-tenth of an angstrom, about the size of a single atom. In a low-Reynolds-number world, lazily performing the backstroke over the course of a year gets you as far as if you complete the stroke in one second.
When inertia no longer matters, terms like mass, acceleration, and force lose their meaning. Statements like Newton’s second law (force equals mass times acceleration) are moot. Newton’s second law for a bacterium is zero equals zero. There is no such thing as acceleration, since bacteria attain their steady swimming velocity nearly instantly upon beginning to swim, and lose it instantly when they stop. Newton’s first law, that objects in motion remain in motion until acted upon, becomes absurd; all bacteria know is that if you are not swimming, you’re standing still. No microorganism gets to move for free. Newton’s third law, that every action has an equal and opposite reaction, becomes something of a tautology, for there are no longer any actions.
In bacteria-world, physics is better described by Aristotle, who denied the existence of inertia.
I was surprised by the ability of bacteria—which their discoverer, van Leeuwenhoek, called “cavorting wee beasties”—to break one of the cornerstones of classical physics. Seeing this, the head of the group I was shadowing guided me to a set of articles written in the 1990s by Nobel Laureate physicists.2,3 The articles proved that the physics governing swimming microorganisms were identical to the physics governing the quantum mechanics of certain subatomic particles. They even described something simple and concrete—the speed and trajectory of a certain alga—with gauge theory, one of the buzzwords with which I had been inculcated as an undergraduate. The fact that bacteria are governed by a gauge theory is precisely what allows them to move without forces and circumvent Newton’s laws.
That was another big lesson for me, not only in physics and mathematics but in sociology and identity. I determined that physicists are not constrained to study certain objects, but rather apply certain tools and modes of thinking to any kind of object in any field of interest. Gauge theory was not just for quantum mechanics, it could also be for microorganisms and other objects closer to our own size.
I wasn’t thinking about my research with pen and paper—I was feeling it with my arms, hands, fingers, feet.
With immense help from the group at UCSD, I got into a Ph.D. program at Brown University. My new colleagues appropriated beautiful tools from theoretical physics to study macroscopic topics such as swimming, the wrinkling of window drapes, and the formation of trees and bones. Learning to recognize structures of theoretical physics in unusual places prepared me for my discovery that, like microorganisms, human beings are also capable of transcending Newton’s laws.
In my first year at Brown, I started learning tai chi to dissipate the stress of graduate school. I’d become obsessed with the problems I was working on, and had become like a cartoon scientist who manically pulls out his hair whilst staring at an immense blackboard filled with scribbles. Tai chi advertised the ability to let go, as well as a certain mysticism and appeal to the athletically inept.
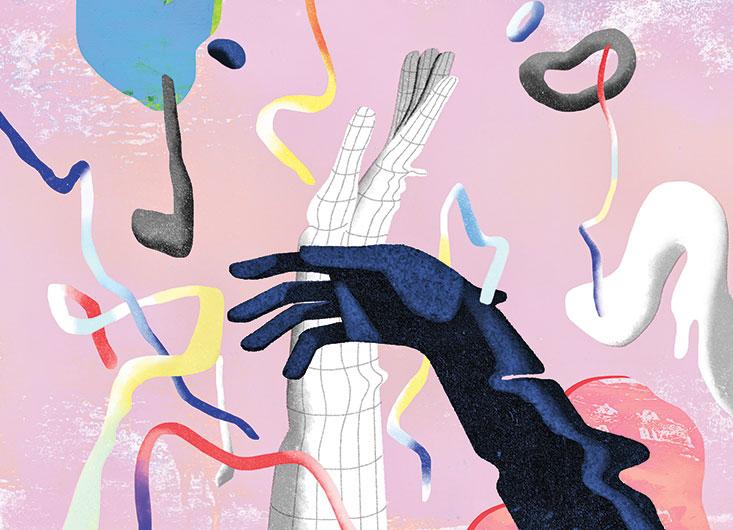
During the initial training, I did indeed learn to relax and forget about my work. But I received an entirely different benefit when I was introduced to the common two-person tai chi exercise known as tuishou, translated as “Push Hands” (or “Pushing Hands”). It can be performed either collaboratively, for the purpose of relaxation, or competitively. The objective of competitive Push Hands is simple: Competitors face one another and attempt to unbalance each other without the use of strikes, grabs, and without pushing below the waist or above the collarbone. The rearrangement of the two competitors is expected to be smooth and continuous, lacking the muscle-against-muscle overtures of other grappling disciplines.
The key to Push Hands training is learning how to not seize up when muscled, but rather loosen and lead an opponent “into emptiness”: manipulating the opponent’s alignment so that their incoming push or pull is redirected safely away from the practitioner’s center of mass. In the beginning stages it’s difficult to identify which components of the body are tight and which are loose, even in oneself—it can take years of studying and practicing tai chi routines to do so. This is an absolutely essential component of training, as any tension provides a direct line to one’s center of mass: It’s like having a steel rod (the tight limb or joint) precariously attached to a leaning structure (the torso). Because the rod doesn’t stretch or bend, anybody can pull the structure down by pushing or pulling on the rod.
Next, a prospective Push Hands competitor must learn to recognize these tightnesses in another person by touch and by sight. This is normally facilitated by pre-arranged, cooperative Push Hands drills which train the skill of ting, which means listening—learning to interpret an opponent’s intention and body alignment haptically through the points of mutual contact. The value of this skill is so great that participants are discouraged from ever breaking contact.
Push Hands challenges you to move and deform your body to dissipate incoming force. No force means no inertia. When there is no inertia, there is an ambiguity in defining terms related to mass. Concepts such as a “center of mass” become ill-defined. If you cannot feel a mechanical connection to your opponent’s center, you can’t say for sure where their center is—it could be in their head, their left pinky, or anywhere else.
All of this left me overwhelmed with a sense of déjà vu. The recognition of something familiar peeking out from behind the mask was immediate, but it took me a while to process, because for the first time I was not thinking about my research with pen and paper—I was feeling it with my arms, hands, fingers, feet. Finally, I realized: Bacteria were masters at tai chi.
Like an expert tai chi practitioner, bacteria also effectively lack a center of mass. If you are a bacterium and crash into another bacterium, the motion is the same whether you think the center of mass is in your head or your flagellum. When two bacteria are in contact they stick and adhere, and move around without exerting a force on one another. Sometimes to neutralize these forces they need to remain together for very long times, almost indefinitely. Somehow the bacteria “know just how to move” in order to not exert force on one another. They are masters of neutralization.
They are also, like Push Hands practitioners, doing the seemingly impossible: neutralizing every force, while still achieving some kind of motion. They speed up and slow down, and change directions on a dime. Newton forbids this to happen in the absence of forces.
This discourse between force and motion taught me the one difference between bacterial swimming and Push Hands. In Push Hands we eventually exert large forces after gaining an advantageous position. Similar strategies can be seen outside tai chi, like Muhammad Ali’s “rope-a-dope” technique of adhering to and riding out punches using the elasticity of the ring ropes to dissipate the incoming energy, which leads to huge forces in the subsequent counter-punch. In a phrase, the bacterium-like motion of the practitioner was to be abandoned precisely when it would maximize an advantage.
For a bacterium, there is no such thing as acceleration.
This led me to wonder—did microorganisms stand to learn anything from tai chi practitioners? How would they behave if they, too, were suddenly allowed to exert force in a timely and strategic manner? To understand this, I had to introduce them to some new physics; the gauge theory of swimming which at first had so excited me was now the chief obstacle in teaching my wee beasties to use force on one another.
This unexpected motivation from martial arts became a major thrust of my doctoral research. I set out to study novel environments for bacteria in which their physics are slightly different. In these environments—like simple fluids in unusual geometries, or unusual fluids in simple geometries—bacteria could do useful work for humans, including assembling and towing small batches of medicine or powering tiny engines. This becomes possible when we break up the conventional physics in a pre-programmed way, much like the Push Hands practitioner breaks the rules of neutralization when the advantage has been won.
Ideas like these are now percolating in many directions and will eventually lead to the fabrication of useful microscopic devices. The ability to trigger forces which allow bacteria to separate from objects after sticking and adhering could let them tow cargo, for example a payload of chemotherapeutic molecules directly to a tumor, delivered without bombarding healthy tissue. (Bacteria are capable of separating themselves without our help, but the mechanism is a bit obscure and involves secreting a lot of different chemicals.) Or small swimmers (natural or manufactured) could manipulate and assemble particles of medicine or other small molecules in intricate patterns, even at a distance, due to the flows they create when they exert force. A bacterium could, in this way, not only assemble anti-cancer medications from some basic ingredients, but also carry them to a tumor, release it, and repeat the process until therapy is complete.
Studying tai chi did more than guide my research—it transformed how I do research. Western philosophy tends to separate the universe into the observer and the observed, with little interaction between the two. Seeing bacteria and tai chi mutually inform one another gave me a perspective on a more egalitarian way of doing physics, by focusing on connections.
There is a fair amount of naiveté in finding connections between bacteria and Chinese martial arts. But this kind of naiveté is central to how theoretical physics is done, and giving in to it for a moment led me to something deeper. I began to write drafts and drafts of something, some crazy idea of physics pedagogy using body techniques. I started ranting to my friends and colleagues about a “physics from the body.”2 To me the connection between tai chi, bacteria, and the mathematical idea of gauge theory seems deeper than random connections between my interests or suggestive little ideas that guided my research to big problems.
I’ve come to appreciate the body as a vector of knowledge, a source separate from the eyes that read books and the ears that hear lectures. In that sense, it is the most accessible vector we have; everyone possesses a body, but not everyone has access to books or lectures. I really believe that obscure ideas which are now thought of as the provenance of mathematicians can be made universally accessible, at least through analogy. If you can connect the reasons why a subatomic particle behaves a certain way to why you and your body behave a certain way, then perhaps it will help you understand the world you live in a little bit better, even if the tiny details get swept under the rug. Or, even better, perhaps it will make you want to understand this world better.
Recognizing this connection has also made me feel like I have a certain place or role, which isn’t necessarily described by the term “physicist.” That word has a rocky and tenuous definition rooted in sociology and popular opinion, and had led me to doubt myself. The kinds of things that scientists are interested in now have long eclipsed such terminology, and the field today deserves a change in mindset and terminology. This new mindset should have a new name: “Thinker” is entirely too pretentious, so I will choose “connector.”
A connector is somebody who notices the connection between things. Connecting is perhaps the most valuable skill for physics, and one possessed by many of its heroes—the folk literature is full of adages about Feynman discovering principles of quantum electro-dynamics by spinning plates in the Cornell dining hall and Einstein discovering aspects of his Brownian motion theory by playing with soup. Scientific discoveries are wrapped in a personal narrative—sometimes it is something as boring as staring at a blackboard for hundreds of hours, but more often there are small breakthroughs that occur in the shower, while bowling, while watching the patterns birds form as they fly.
These small connections rarely make it into publications, are rarely discussed in Nobel acceptance speeches. But in a sense are the most important part of the story not only because they are the most humanizing and accessible, but because they reflect the nature of scientific development. And if that’s true, then our quotidian activities are not so different as we might think from smashing atoms together to guess how the first stars were born. Like yin and yang, they usually go hand-in-hand, whether we notice or not.
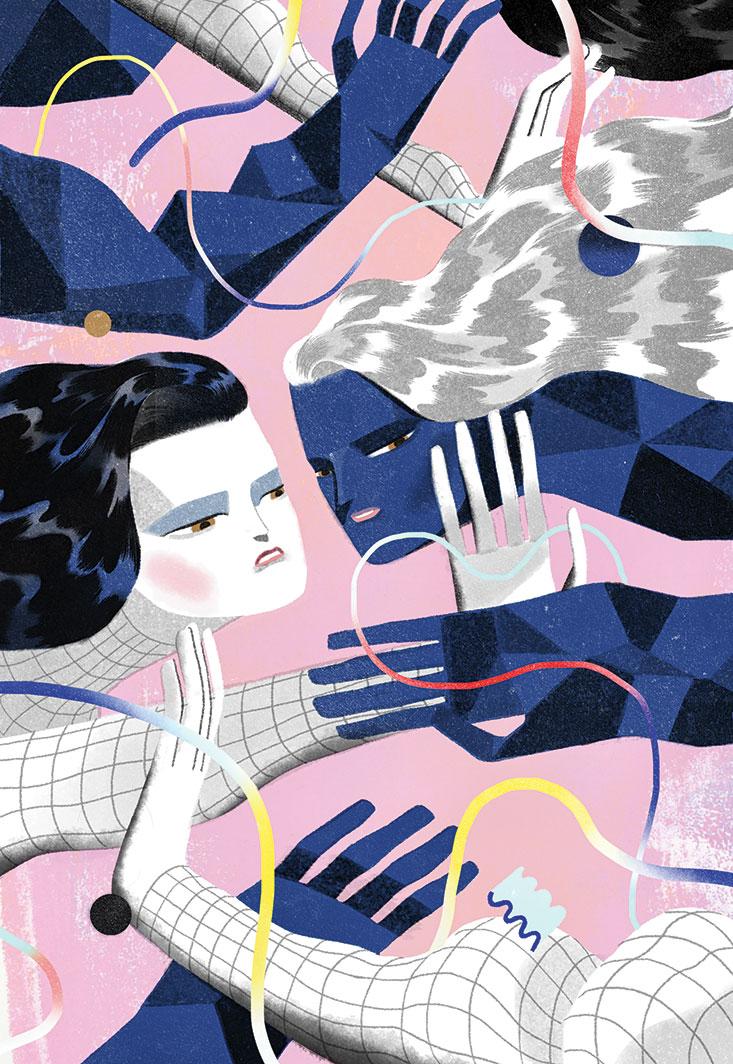
Madison Krieger recently received his Ph.D. in engineering from Brown University and is currently a postdoctoral researcher at Harvard’s Program for Evolutionary Dynamics.
References
1. Purcell, E.M. Life at low Reynold’s number. American Journal of Physics 45, 3-11 (1977).
2. Shapere, A. & Wilczek, F. Geometry of self-propulsion at low Reynolds number. Journal of Fluid Mechanics 198, 557-585 (1989).
3. Shapere, A. & Wilczek, F. Efficiencies of self-propulsion at low Reynolds number. Journal of Fluid Mechanics 198, 587-599 (1989).