In a recent experiment done at the Max Planck Institute for Quantum Optics, in Germany, physicist Alexey Grinin and his colleagues came a step closer to resolving one of the more significant puzzles to have arisen in particle physics over the past decade. The puzzle is this: Ordinarily, when you set about measuring the size of something, you’d expect to get the same answer no matter what you use to measure it—a soda can has the diameter it does whether you measure it with a tape measure or callipers (provided these are properly calibrated, of course). Something must be amiss if your attempts to measure the can return different answers depending on the equipment, yet this is precisely what’s happened over multiple attempts to measure the spatial extent of a proton. What’s potentially at stake is our understanding of the building blocks of reality: the differing measurements could be heralding the existence of new forces or particles.
What does it mean for a subatomic particle to have a measurable “size”? Mathematically, fundamental particles are idealized as point particles, which is to say that, as far as we can tell, they have no meaningfully discernible spatial extent, or substructure, at all. True, all fundamental particles are associated with a quantum mechanical wave packet, which does have a spatial extent that depends on the energy of the particle. Yet these basic bits of Lego are entities whose wave packets you can, in principle, pack into as small a region as you’d like before the very notion of continuum geometry starts, at the Planck scale, to lose meaning. Fundamental particles organize into something analogous to a mini periodic table—consisting of the various force carrying particles, such as photons and gluons (the carrier particles of the strong nuclear force), along with three generations of quarks and leptons and the mass-generating Higgs boson—and can stack together in different combinations to form a zoo of so-called composite particles.
There is less than one in about a trillion chance that the discrepancy could be a statistical fluke.
Perhaps the most familiar and ubiquitous of these is the proton. With at least one in every kind of element, it’s made up of two up quarks and a down quark that dance around each other in a tightly bound orbit maintained by exchanging gluons. This exchange process is so energetic that most of the mass of the proton (or for that matter, most of the material that makes us up) derives from the energy contained in these gluons—a consequence, as Einstein informed us, of E being equal to mc2.
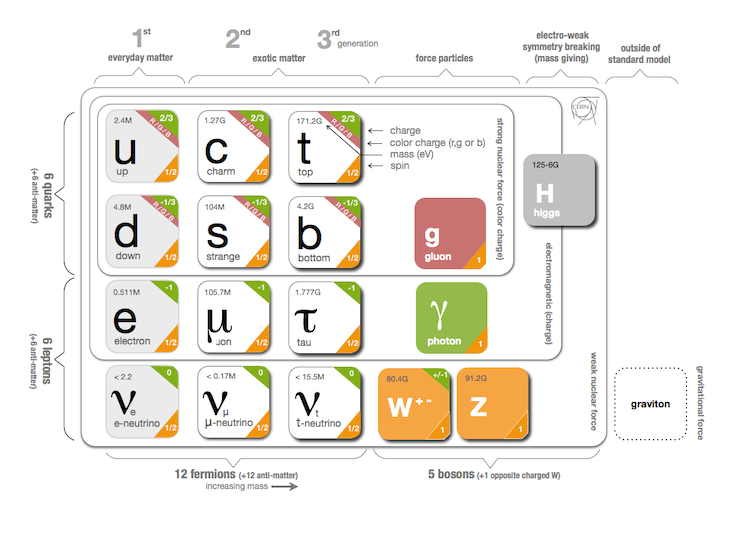
So it’s not meaningless to ask what the “size” of the proton is. The study by Grinin’s team highlights the fact that defining this notion remains a rather tricky affair. And, as we’ll see, their results serve to sharpen the mystery as to why other measurement methods researchers have used previously disagree.
A physicist can reasonably infer a proton’s size from the “charge radius”—roughly the averaged spatial extent of quark orbits inside. This quantity is probed in slightly different ways by electrons and muons (another sort of fundamental particle), when you probe their orbital configurations as they form “bound states” with the proton—atomic hydrogen in the case of electrons, muonic hydrogen in the case of muons. Because muons are about 200 times heavier than electrons, their lowest energy orbital configurations are much more tightly bound around the proton than are electrons in atomic hydrogen. Consequently, the differences in the energies of various orbitals in muonic hydrogen are much more sensitive to the proton’s size as well as being more “high pitched” than that of regular atomic hydrogen.
In other words, similar to how plucking a guitar string at a given tension produces a much higher note were we to fret it open, or at 1/200th its open length, the typical frequencies of the radiation emitted by transitions in muonic hydrogen are about 200 times higher than that in atomic hydrogen. These frequencies relate to something called the Rydberg constant—the tension of the guitar string in the analogy—which appears to be one of the potentially more significant sources of uncertainty proton size-wise. Orbital energy levels depend on both this constant and the charge radius of the proton.
Proton-size measurements didn’t conflict for decades. Different methods—like measuring the radius by observing electrons orbit within hydrogen atoms, or by scattering energetic electrons off of unbound protons—had converged on a value of 0.875 (give or take 0.006) femtometers. That’s a little less than a trillionth of a millimeter. That convergence was disrupted in 2010, when a paper came out titled, “The size of the proton.” As the researchers reported, measurements involving orbital configurations in muonic hydrogen returned a value of 0.842, give or take 0.001 femtometers. This may not seem like much of a difference, but it’s the accompanying error bars that matter. The measurements are, individually, so precise that their disagreement is over seven standard deviations—there is less than one in about a trillion chance that the discrepancy could be a statistical fluke.
There are only two possibilities for the anomalous result if the equipment used in the experiments and their calibrations all check out after careful scrutiny. Either some combination of physical constants, which researchers assume in order to experimentally infer the proton charge radius, isn’t known as accurately as we thought, or there is something different about the way muons interact with protons, compared to electrons, that renders particle physics incomplete.
The latter possibility, if substantiated, would, of course, cause a flurry of excitement among theoretical physicists to say the least, as it could imply the existence of new forces and particles. Not only would it reshape our understanding of the universe, it would represent a throwback to the days when physicists discovered particles (such as the muon itself) using equipment that could fit on a proverbial tabletop.
Over the past few years, various teams have been attempting to get to the bottom of the matter by looking at different orbital transitions in atomic hydrogen that are sensitive to different combinations of the Rydberg constant and the charge radius. A 2019 measurement by a group of researchers at York University in Canada looked at a particular orbital transition that was independent of the value of this constant, finding a value of 0.833 ± 0.010 femtometers, consistent with the smaller value obtained in muonic hydrogen.
Grinin’s team went a step further. They used a technique known as frequency comb spectroscopy. It involves pulses of laser light that are a superposition of equally spaced frequencies—a ruler in frequency space if you will—that allowed them to look at two different orbital transitions in atomic hydrogen sensitive to two different combinations of the proton size and the Rydberg constant. This permitted them to determine both with unprecedented accuracy. The technique reduced, to only about one part in ten trillion, the observational uncertainties in the frequency of light these transitions emitted—a staggering degree of accuracy by any standard.
Not only did Grinin’s team find a value for the charge radius of the proton consistent with the value obtained in muonic hydrogen, they inferred a much more precise value for the Rydberg constant. This accounted for some part of the discrepancy seen in other measurements in atomic hydrogen (which presumed a less accurate value).
It thus appears that the experimental value of the proton charge radius Grinin’s team obtained in atomic hydrogen is converging on the smaller values for the proton charge radius other researchers initially obtained in muonic hydrogen. The smaller value has by now even been adopted as the official value on the National Institute of Standards and Technology CODATA list of recommended physical constants—the official almanac for nuclear and atomic chemists and physicists.
Although this convergence, based on the continued refinement of experimental techniques, did not deliver the new physics some may have been hoping for, even the most despondent theoretical physicist can acknowledge the experimental artistry that seems to be bringing the matter closer to conclusion. What remains unresolved is the reason why measurements, relying on different spectroscopic methods in atomic hydrogen, return different values for the charge radius of the proton. The mystery, and along with it, the diminishing hope of particle physicists, endures for the time being.
This was enough motivation for a team of theoretical physicists, led by Cliff Burgess at the Perimeter Institute, in Canada, to systematically catalogue all possible sources of theoretical uncertainty in atomic spectroscopy over a series of papers. By isolating the ways in which new forces and particles might leave a tell-tale signature, they’ve thrown the gauntlet firmly back to the experimentalists. Future experiments, as always, will be the ultimate arbiter in this matter.
Subodh Patil is an assistant professor at the Lorentz Institute for Theoretical Physics at Leiden University. He tweets on occasion at @_subodhpatil.