The universe is not just expanding; it’s accelerating. When cosmologists first realized this in 1998, it was alarming. The attractive gravitational pull of matter should cause the expansion to slow down. But this isn’t what we see. The galaxies in the universe are not only moving away from us—they’re moving away faster today than yesterday.
We’re not sure what is causing the expansion to accelerate, so we’ve called it “dark energy.” Our best theories of what matter is and does give us a candidate for what dark energy might be—something called vacuum energy. It’s the energy in a region of space, contained in the electromagnetic field and its cousins, even when there are no particles. But when physicists attempted to calculate the amount of vacuum energy, they got a shock. The answer was about 10120 times larger than the actual value learned from observation. This is a problem.
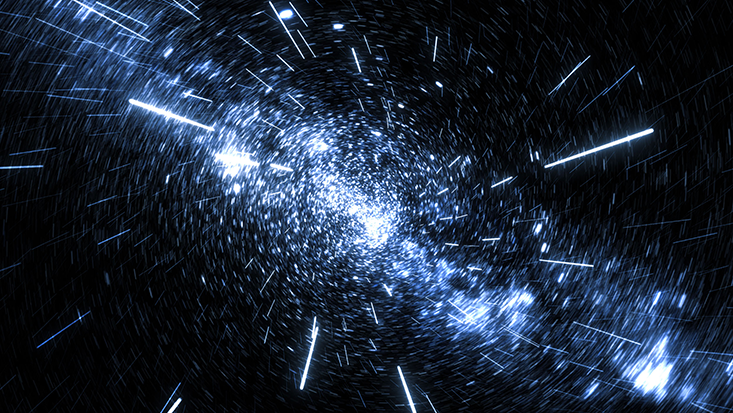
Even prior to the observational confirmation of dark energy, Steven Weinberg, the Nobel Prize-winning physicist, had wrestled with the theoretical overestimate and suggested a remarkable solution. He noted that the amount of vacuum energy can vary from place to place, depending on how all the different fields in the universe add and subtract. In our region of space, the amount of vacuum energy is minuscule, requiring all these fields to cancel one another out to an incredible degree. That seems highly improbable, but in any large enough system, even the most improbable event will occur somewhere. In the vast majority of cosmic regions, the excess of dark energy throws off the balance of forces that enables galaxies, stars, planets, and people to form. We see an improbably precise cancellation of known and unknown fields because we couldn’t exist otherwise.
We have been testing Weinberg’s idea in computer simulations, and we’re finding that galaxies are more robust than we thought. They are controlled more by their internal balance of forces than by the external influence of dark energy. If that is true, then the amount of dark energy we observe can’t be explained as a selection effect, and we are back to square one in our understanding of dark energy.
Our cosmic environment is the result of a delicate balance of cosmic forces—gravity and pressure, cooling and heating, expansion and collapse. The final product, when all these pushes and pulls come into balance, is our Milky Way galaxy, where stars form in a rotating disk of gas and a diffuse halo of dark matter.
In any large enough system, even the most improbable event will occur somewhere.
To understand the multistage process whereby galaxies and stars form, our simulations subdivide matter into small chunks that are pushed around by gravity, pressure, and other forces. We place these chunks in an expanding universe and calculate their gravitational pull on each other. The first balance we must analyze is between gravity and expansion; one pulls material together, the other draws it apart. Gravity will win in regions that are sufficiently dense. Those parts of the universe will collect yet more matter from their neighborhoods. The rich get richer, the big get bigger, and our initially smooth universe develops structure.
Gravity assembles matter into roundish “haloes.” These, too, achieve a balance, this time between gravity and the random, chaotic motion of matter. To disrupt this balance and allow galaxies to take shape, we need gas cooling. Gas in the roundish haloes is hot—so hot, in fact, that it glows. This glow leaks away thermal energy, which can upset the balance. Gravity eventually wins, and the matter collapses until it reaches its final equilibrium configuration, supported by rotational motion. As particles are swept up into the forming disk, a galaxy is born. Within this disk, stars can form.
Ironically, simulations that include the physics discussed so far are too good at making galaxies and stars, much better than real galaxies. The missing piece is feedback: As stars form, they pump energy back into their neighborhood, heating the gas. When large stars run out of fuel, they explode, which can send matter back out of galaxies. Stars inhibit star formation, allowing the balance we observe to be struck.
With this physics, and weeks to months on thousands of linked computers, these simulations reveal a condensing web of cosmic structure, with dark matter and ordinary matter coming together to produce remarkably realistic galaxies. If Weinberg is right, this process should not work in regions of the universe that have more dark energy.
To see why other regions could fail to form galaxies, let’s trace the matter that is destined to become a galaxy such as our Milky Way. In our simulated universe, this patch of matter first fragments into smaller pieces, some containing small galaxies. The young Milky Way grows both by collecting more gas to make stars and by accreting smaller galaxies. Around the Milky Way today we see long, thin streams of stars, the remains of smaller galaxies, long since shredded.
What happens as we ramp up the amount of dark energy? The accelerating phase of the expansion of the universe begins earlier. In the competition between gravity and cosmic expansion, the latter starts winning sooner and eventually brings the formation of structure to a grinding halt. Smaller galaxies that would have fallen into the young Milky Way are carried away by expanding space. The gas that would have fueled star formation never comes within the galaxy’s gravitational pull. The young Milky Way becomes isolated and impoverished. With limited fuel, it burns out and enters senescence.
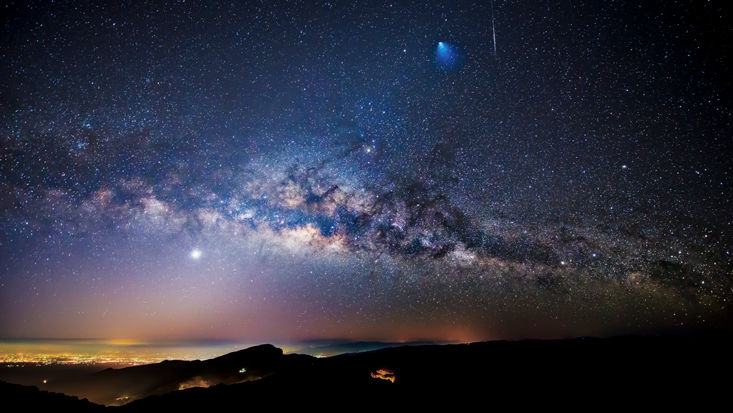
Zooming out, we see a universe sparsely populated by small galaxies, with the intervening gas stretched too thin to make stars and too far from the nearest galaxy to be drawn in. The universe’s structure-forming epoch, its creative phase, is over.
If we continue to ramp up the dark energy, the formation of structure freezes before even the smallest galaxies form. None of the matter in the universe manages to form into galaxies, stars, planets, or people. The entire universe is stillborn—a diffuse, expanding gas of particles that very occasionally bounce off each other, but do little else. There is no life, because there is no structure or complexity at all.
On the face of it, the effects we find support Weinberg’s hypothesis. But there is a twist in the saga.
In our universe, star formation was most efficient a few billion years before dark energy started driving accelerating expansion. By the time galaxies were isolated by cosmic acceleration, star production was declining anyway. Stellar feedback is the major reason why the universe doesn’t form stars very efficiently. Dark energy is only a secondary villain.
As a result, adding even 10 times more dark energy doesn’t make much of a difference. Only with significantly more dark energy—we’re still working out how much more—does cosmic acceleration really kill star formation.
We haven’t yet published our findings, but if they hold up, Weinberg’s argument looks shaky. Suppose there are other regions of the universe with more or less dark energy than we have. Essentially, each region picks a number between zero and 10120, on a scale where our own region picked 1. Now imagine taking your survey clipboard around to check on the lifeforms that evolved in those regions; if you meet one, ask how much dark energy they see. Most regions are dead, of course, which explains why there is no one to tell you, “My region picked 1030 or 1090.” But there are plenty of observers who see 10 or 100 or more, in which case we might wonder why we are the outliers who see a value of 1. If the theory says “expect about 100,” but we see 1, we cannot explain our value as a mere selection effect.
It’s early days. We’re still analyzing. Our computer continues whirring. But, on balance, perhaps we should keep looking at other options to explain dark energy.
Luke A. Barnes is a postdoctoral researcher at the Sydney Institute for Astronomy at the University of Sydney. He focuses on galaxy formation. His book with Geraint Lewis, A Fortunate Universe: Life in a Finely Tuned Cosmos, was recently published by Cambridge University Press.