Play Video To view the video, click the “play” icon above.
It was just a regular day at the Yellowstone Club in Montana, as far as the weather was concerned. No heavy snowfall or vicious winds were preventing snow mechanics engineer David Walters and five of his colleagues from doing their field work. Walters’ academic advisor had even chosen to do some skiing nearby. But just as the team were about to leave, Walters heard a cry. An avalanche had begun to tumble down around his advisor, Daniel Miller.
Thankfully, it was a small one, and slid only about 30 feet down the mountain. But what was remarkable was that it had happened at all. The slope Miller had been skiing down had a gentle, 22-degree incline. According to the Swiss Federal Institute for Snow and Avalanche Research, one of the largest research institutes of its kind, inclines less than 30 degrees are considered safe. “We always have this 30-degree number in our head,” Walters told me. “A 22-degree slope—we almost consider that a golf course!”
The 30-degree rule of thumb is just one of many qualitative characterizations of the ultimate domino in the snow. Avalanche forecasting relies on subjective interpretation, both of field data by rangers, and by skiers of the five risk categories that appear in American forecasts: low, moderate, considerable, high, and extreme. But new approaches to the science of snow are promising to dramatically increase forecasting accuracy.
At the top of this page, filmmaker Abby Kent tells the story of two scientists working in this field in her short documentary, Avalanche Engineers. David Walters and Tony Lebaron work in two very different laboratories: the slopes of the American Rocky Mountains, where the tools are ski poles and measuring sticks, and the “subzero lab” at Montana State University, where the tools are pressure sensors and three-dimensional CT scanners. In the documentary, as in my interview with Walters, below, we discover just how deep avalanche science can go.
What causes avalanches?
First, the snowpack structure needs to be suitable. That means having some sort of weak layer or weak interface in the snowpack. When we have these fairly warm, sunny days in the mountains—below freezing, but the kind of days that everybody likes skiing around—the solar short-wave energy that the sun is putting out can actually penetrate and warm the snowpack, a couple of centimeters deep. Simultaneously, there is a cooling effect that acts right on the surface of the snow, which is next to cold air. Imagine the surface of the snowpack trying to get colder while a couple of centimeters down, the snow is trying to get warmer. So we get this difference in temperature. Nature is constantly trying to even out differences in temperature. Since the original, strong configuration of snow grains is so insulating, heat movement from the warm spot to the cold surface is slow but continuous. This movement of heat within the snowpack also takes water vapor from the crystals in the warm spot. The water vapor flows until it hits the cold spot, and then it condenses out, but as ice, into a new grain of snow.
After the snowpack settles, we simulate a new storm coming in, or some windblown snow coming in, creating a slab.
This rearranges the snow crystals within a 2-centimeter zone. We’re not adding any snow; we’re not taking away any snow; but we’re taking the structure that’s there and using vapor movement resulting from that difference in temperatures to rearrange the microstructure of the snow into a more efficient pathway for heat to move. Unfortunately, this also produces a mechanically weaker structure. Then that weak layer is eventually buried by some stronger layer, which we call a slab. The slab is a hard, cohesive layer of snow that can be very large, spanning an entire face of a mountain. And now it’s resting on top of a weak foundation. It’s like building a house on a foundation of potato chips.
Finally some trigger, like a skier or a snowboarder or a snowmobiler, puts enough force or stress onto the snowpack to break the weak layer, and causes that slab to start riding over the top as it goes downhill. Sometimes a slab avalanche can break several hundred meters wide.
Is a slab necessary for an avalanche?
Most of the deadly avalanches that we see are slab avalanches. If the snow on the weak layer hasn’t become cohesive enough, we can still get loose snow avalanches. They can still generate a lot of energy, but because they don’t stick together, you’re not going to be going for as much of a ride in them.
How do you start researching avalanches?
We actually start a lot of our work in the field, observing the conditions that form weak layers. We have a couple of field stations down near Big Sky, Montana, at one of the private ski areas, called the Yellowstone Club. The ski patrol there is gracious enough to go out on a daily basis and basically, collect notes for us on what’s going on with the snowpack. Once we figure out the days that create the weak layers, we can take those sets of conditions and come back to our lab.
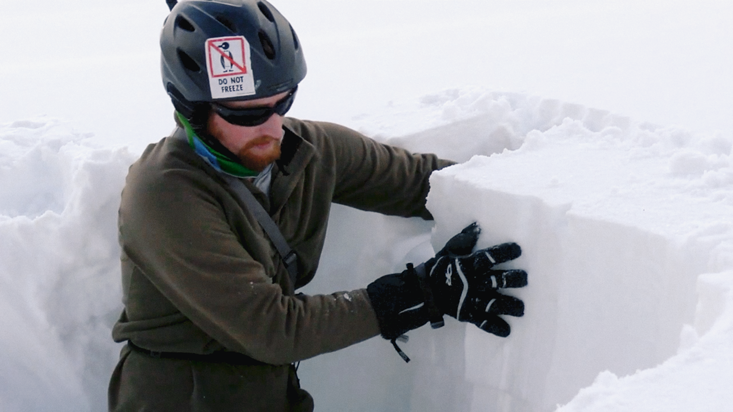
What do you do in the lab?
We recreate the conditions we see outside. We can control the amount of sunlight we have in the lab with a metal halide lamp, and recreate sky conditions by controlling the temperature of the lab ceiling and the air temperature. From there, we just stick a sample of snow underneath the lamp and run a full day’s worth of sun on it. While this is going on, we’ll grab little samples of the snow and analyze its microstructure in our micro CT scanner, which produces a 3-D picture of the snow’s structure. After the lamp gets turned off and the snowpack settles, we simulate maybe a new storm coming in, or some windblown snow coming in, creating a slab.
When the snow is easier to fracture, avalanches are much more likely to occur with lighter and more sensitive triggers.
To simulate an avalanche, we embed a metal frame inside the upper slab layer of snow so that we have something to push on, and isolate a column so that we know exactly how much snow we’re dealing with. Then we push on the snow until it fails. This tells us what the effective mechanical properties were of both the slab layer and the weak layer.
Then this is where I get to sit in front of my desk for far too many hours, looking at micro CT scans or 3-D pictures of the microstructure. We use various models to try to understand how and why we got the effective properties that we did.
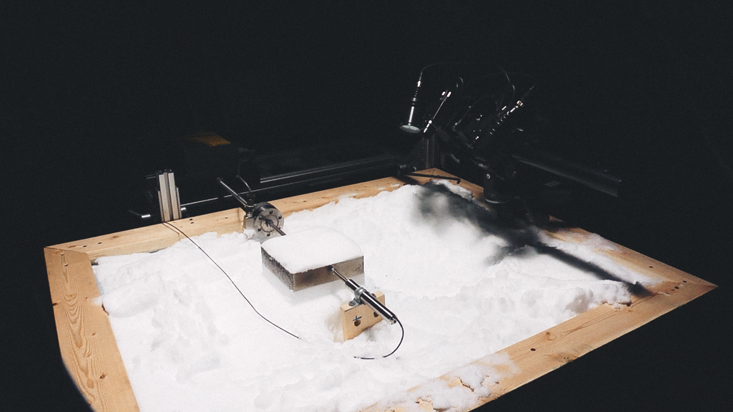
Why are the bonds between grains of snow important?
Bonds are what join two or more individual snowflakes or snow grains together. If we have two grains of snow that are half a millimeter in diameter each, I might call the area that joins those two grains together, maybe a quarter millimeter in diameter, a kind of bridge or bond. It’s all one continuous piece of ice, but there is this restriction or narrowing that is a bonded area. Rounded grains tend to form a cohesive snowpack, and are usually associated with slabs. The orientation of bonds are random in that case, without a preferred orientation. When we get into faceted layers of snow, the bonds can start to arrange themselves in a particular pattern. We think that when the bonds between snow grains are oriented randomly, it is difficult to propagate a fracture through the snow—there is no clear, obvious path within the microstructure. However, in a weak, faceted layer of snow, the patterned alignment of bonds can provide a quick, straight, and easy path for fractures to propagate, thus leading to total failure of the layer with far less energy. When the snow is easier to fracture, avalanches are much more likely to occur with lighter and more sensitive triggers.
What effect will your work have on forecasting?
If we can predict the mechanical properties of snow, and how much weaker or how much stronger snow has gotten from its initial state, it can help with forecasting. One of my colleagues, mechanical engineering graduate student Patricia Curley, is working on a very cool model that takes a digital terrain map, like Google Earth, and weather conditions to model a snowpack. In this model, we can track the sun moving through the sky, see how shadows affect certain slopes, and know what the temperatures are, both on the surface of the snowpack as well as underneath the surface. Her model can map the locations where we get these critical temperatures, or temperature gradients that form weak layers. We can then start to forecast weak layers for specific mountains—not just guess at how weak those layers are, but be able to put a number on it. This is maybe 50 years down the road, or 100 years, but I think we can get there. We can say, “Remember that weak layer that was formed a week ago, and then the storm that came in? Well that storm loaded that weak layer to within 50 percent of its strength.” So maybe the weight of a skier might not trigger that avalanche, but a snowmobiler might.
Yvonne Bang is an Assistant Editor and Video Production Manager at Nautilus.
Abby Kent is a freelance filmmaker and animator. @AbbyKentMedia