In the United States alone more than 120,000 people are waiting for organ transplants, and many will die before their turns come. What if they didn’t have to wait, because doctors could print out replacement organs on demand?
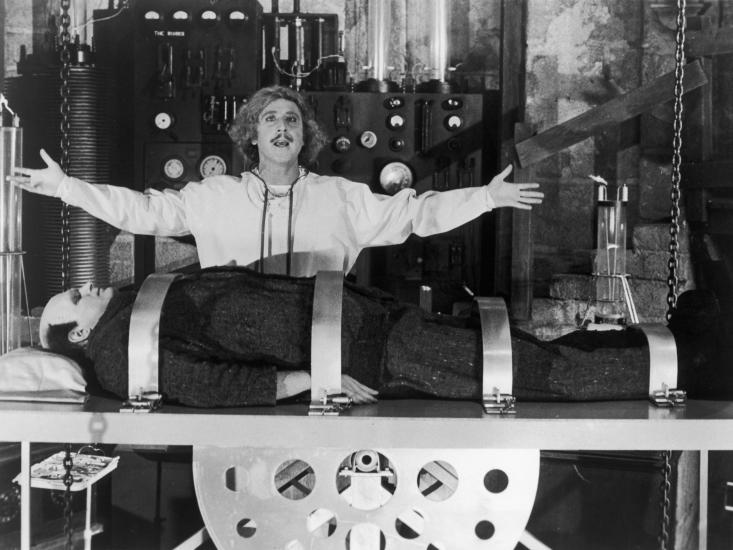
That’s the ultimate goal of bioprinting, a seemingly sci-fi spinoff of the burgeoning industry of 3D printers. If all goes according to plan, a medical technician will eventually be able to cast living cells into a complex three-dimensional shape that replicates the anatomy and action of a human liver, for example, or a beating human heart.
Today’s bioprinters can already produce chunks of functional human tissue, which is a pretty remarkable feat of biological manufacturing. But don’t expect an assembly line for full-sized organs any time soon. Scientists’ major challenge is scaling up: They must create tissue with enough structural integrity to hold an entire organ together, and find ways to knit that synthetic organ into the body’s network of blood vessels.
This work has powerful cultural resonance. Nearly 200 years ago Mary Shelley gave us Victor Frankenstein, the bold scientist who assembled a creature from human body parts. Frankenstein presented a cautionary tale of what can happen when a mere mortal tries to play God and has the hubris to think that human handiwork can match nature’s masterpieces. Now we have a different narrative. The promise of creating life-saving body parts shows the benefits of, if not playing God, at least playing Victor Frankenstein. Bioprinting has turned mad science sane.
Let’s borrow from Frankenstein to show how bioprinting works. To understand how a standard (non-biological) 3D printer works, imagine that you want to sculpt the neck and head of Frankenstein’s monster out of toothpaste. Squeezing the tube to extrude a steady line of goo, you first put down a layer in the shape of his neck. Then you go over the shape again to put down a second layer and make the neck rise higher. Of course this wouldn’t work for long: With toothpaste, your project would soon collapses into a sticky mess.
But inside a 3D printer, your Frankenstein head would turn out considerably better. A printer nozzle would extrude a precise jet of material, often a plastic or molten metal, and quickly harden each layer before starting on the next. Based on the instructions from a computer file, the printer would build the neck and then the head, slice by slice.
To print a liver, in theory, a 3D printer would follow instructions describing the internal structure of the organ, in which clusters of liver cells are interwoven with blood vessels, bile ducts, and nerves. The printer nozzles would squeeze out the proper types of cells in the proper positions, layer by layer, to build the complete 3D organ. But cells on their own don’t adhere to each other; they need to be embedded in a material that gives the organ structural integrity. That mix of living cells and binding material is the printer’s “bioink.”
Making an effective bioink is decidedly tricky. The material must be fluid enough to flow out of a printer nozzle, but must solidify very quickly once it’s been printed. To make bioprinting feasible, each layer must harden before the next layer is added, so scientists use materials that set in response to catalysts like a temperature change or a flash of ultraviolet light. However, the hardening process must be carried out without damaging the living cells in the bioink.
Once a researcher has a workable bioink, it’s stashed in cartridges in the bioprinter. Just as your inkjet printer might have multiple cartridges holding inks of different colors, a bioprinter could have cartridges containing inks suffused with different kinds of cells. The bioprinter then goes into action, moving its nozzles around inside a fabrication chamber, following precise instructions on when to squirt out each ink. The nozzles do their first pass, the bioprinter hardens that layer, and the process repeats.
Making an effective bioink is decidedly tricky
The best bioinks invented to date are still far from capable of building a large organ like a liver; scientists haven’t yet found a gelatinous material that can be squeezed out of a nozzle, hardened enough to give the shape structural integrity, but kept in a state that allows living cells to survive.
One solution to this conundrum comes from researchers at Carnegie Mellon University, who found a different way to support the tissue during the printing process. In their technique, which they described recently in the journal Science Advances, they printed their tissue inside a chamber filled with a different kind of gelatin. As the bioink accumulated and began to form the intended shape, the surrounding gelatin didn’t fuse with the printed object but instead cradled it gently. When the object was finished, the whole container was warmed up and the heat-sensitive support gel melted away, leaving the intact object behind.
The Carnegie Mellon team printed out complex anatomical shapes, including a replica of an embryonic chicken heart and a miniature human brain. These objects were small, on the centimeter scale, says lead researcher Adam Feinberg. However, he can imagine several ways to use this printing system to scale up. Right now, the dimensions of the printing machine his team built limit him. “But we could fill up a big bucket with the gelatin slurry and print inside of that,” Feinberg says. They’d just need to rig up a system to lower the nozzle deep into the bucket, where it would start building the base of a large object. “We haven’t done this,” he says, “but I can’t see any reason why it wouldn’t work.”
Another approach: Feinberg can envision a printer with many nozzles that emerge not only from the top of the machine, but its sides as well. This method would allow printers to build a large object quickly by extruding material from multiple directions at once. The speed of printing is important, Feinberg says, because cells don’t live long without a supply of nutrients. “You have about a two-hour printing window before you’re going to have a lot of cell death,” he explains. To pull off this multi-directional technique, researchers would have to invent the machine and write the software to control it, but Feinberg doesn’t see these tasks as showstoppers.
Creating blood vessels throughout a manufactured organ is another challenge. Some research groups are printing objects composed of both bioink and a special “fugitive ink” that mimics the twisty shapes of internal blood vessels. Once the printing is complete, a temperature change causes the fugitive ink to turn from gel to liquid, which then drains out of the object to leave empty spaces in the shape of blood vessels. A second approach involves printing the cells that make up the walls of blood vessels, and encouraging them to grow into complicated networks of tiny capillaries.
There’s a lot of work to be done, and most experts think full-sized organ printing is decades away. But today’s bioprinting technology can already provide significant medical benefits. Drug companies can use 3D scraps of human heart or liver tissue to test new pharmaceuticals for toxicity. These tests are expected to be far more predictive than both animal tests and those that use two-dimensional smears of human cells in Petri dishes.
“There’s agreat demand for this screening,” Feinberg says. Many new drugs fail because of side effects to the heart or liver, he explains, and drug companies often don’t find out about those side effects until they reach clinical trials in humans. One of the first bioprinting companies, the San Diego-based Organovo, already offers drug-testing services with its 3D-printed liver tissue.
Feinberg also anticipates printing scraps of cardiac tissue as patches for the damaged organs of heart attack patients. Doctors could use MRI scans to determine the exact shape of the damaged tissue, print out the patch using cardiac cells derived from the patient’s own body, and graft it on.
Of course, government regulators will have to deem this procedure safe. But if they do sign off, we’ll be one step closer to printing out entire hearts for desperate patients. It seems a safe bet that patients in dire need of replacement organs would see that end-goal as a good resolution to Frankenstein’s story. When it comes to their health, most people don’t care where their remedies come from—they just care if they work.
Eliza Strickland is an associate editor for the science and technology magazine IEEE Spectrum.