An Introduction to the Black Hole Institute
Fittingly, the Black Hole Initiative (BHI) was founded 100 years after Karl Schwarzschild solved Einstein’s equations for general relativity—a solution that described a black hole decades before the first astronomical evidence that they exist. As exotic structures of spacetime, black holes continue to fascinate astronomers, physicists, mathematicians, philosophers, and the general public, following on a century of research into their mysterious nature.
The mission of the BHI is interdisciplinary and, to that end, we sponsor many events that create the environment to support interaction between researchers of different disciplines. Philosophers speak with mathematicians, physicists, and astronomers, theorists speak with observers and a series of scheduled events create the venue for people to regularly come together.
As an example, for a problem we care about, consider the singularities at the centers of black holes, which mark the breakdown of Einstein’s theory of gravity. What would a singularity look like in the quantum mechanical context? Most likely, it would appear as an extreme concentration of a huge mass (more than a few solar masses for astrophysical black holes) within a tiny volume. The size of the reservoir that drains all matter that fell into an astrophysical black hole is unknown and constitutes one of the unsolved problems on which BHI scholars work.
We are delighted to present a collection of essays which were carefully selected by our senior faculty out of many applications to the first essay competition of the BHI. The winning essays will be published here on Nautilus over the next five weeks, beginning with the fifth-place finisher and working up to the first-place finisher. We hope that you will enjoy them as much as we did.
—Abraham (Avi) Loeb
Frank B. Baird, Jr. Professor of Science, Harvard University
Chair, Harvard Astronomy Department
Founding Director, Black Hole Initiative (BHI)
Black holes are the most extreme objects in the universe. Pitch black, with masses as much as a billion times that of our sun and with the strength to anchor whole galaxies, such extreme objects call for equally extreme methods to study them. Indeed, scientists go to great lengths to pick up whatever signals they can from these invisible beasts: telescopes launched into space to detect X-rays that are emitted from black holes but can’t pass through our atmosphere; kilometers-wide neutrino detectors placed on the floor of the Mediterranean Sea, catching elementary particles coming from the black hole births; and over $600 million spent building the Laser Interferometer Gravitational-Wave Observatory, which offers the first opportunity to observe black hole activity directly.
But what if black holes could be studied in a more modest way, without fields of telescopes or millions of dollars in equipment? What if, rather than chasing them in the wild, scientists could build them in the lab and then study their behavior up close and in detail?
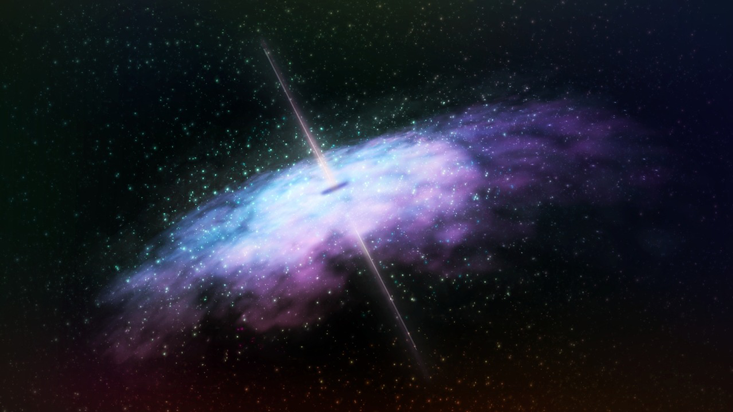
This is the world of analogue black holes. Here, scientists reproduce aspects of the incredible physics of black holes in labs and on tabletops, using unassuming materials like glass, water, and clouds of gas. It’s an approach that, in bringing black holes down to earth, offers an important complement to the traditional methods of galactic observation, and may even lead to the engineering of new, important materials that incorporate some unique properties of black holes.
The logic behind analogue black holes is simple, and it starts with the fact that we have some beliefs about how real black holes work. We know, for example, the equations that govern the event horizon—the place where the pull of gravity becomes so strong that it creates a point of no return for any substance passing through it, including light. We also know of other physical systems that obey these same equations, but which are much easier to manipulate and measure. So if we want to test what happens around the time light passes through the event horizon—but don’t want the trouble of observing it in a black hole light-years away—we can instead set up a system of fluids here on earth that follow the same rules. This system will have its own version of the parts of a black hole, including a point of no return for the waves traveling through it. The observations made in this system can then be mapped back on to their counterparts in black holes. In this way, the equations function like the script of a play, with black holes casting one set of actors and the analogue systems casting another.
The difficulty of detecting such a weak yet important signal is exactly what motivates the use of models.
The inspiration to study black holes through analogues came from a screaming fish. In 1972, physicist William Unruh came up with the story of a fish falling over an unreasonably fast waterfall as a means of describing a black hole. If the waterfall moved at speeds faster than the speed of sound, he explained, there’d be a point at which the screams from a fish that had fallen over it would never be heard from outside the falls. This is because the screams couldn’t move upward faster than the water pushes them down, the same way light can’t break free from the overwhelming gravity of a black hole once it passes the event horizon. While this absurd story started as a mere illustration, Unruh eventually fleshed it out into a proper mathematical argument, showing in a 1981 paper how such “sonic” or “deaf” black holes mapped mathematically onto the real thing. The field of analogue black holes was born.
Exactly which properties of black holes can be explored using these analogues? Hawking radiation—a hypothesized means by which black holes can evaporate—has been a major focus of study, as its existence is both uncertain yet hugely consequential if true. In 1974, Stephen Hawking combined ideas from the largely incompatible theories of general relativity and quantum mechanics to show that black holes may actually emit particles, which over time would cause them to shrink and even disappear. To observe traces of Hawking radiation from black holes would thus do a lot toward bringing these two opposing fundamental theories of physics together. But there is one crucial problem with trying to measure this effect “in the field”: Hawking radiation is so weak any sign of it would be completely wiped out by the cosmic background radiation that permeates the universe.
The difficulty of detecting such a weak yet important signal is exactly what motivates the use of models in most scientific disciplines. In biology, for example, it can be hard to identify the function of a gene in data that is obscured by other factors such as lifestyle differences. So biologists turn to highly controlled laboratory settings, where genetic modifications are known and their impacts can be measured precisely. The same opportunity for control and precision can also drive astrophysicists away from the telescope and into the lab.
This migration to the lab may have paid off. In 2016, after many years of work, experimental physicist Jeff Steinhauer published the results of a miniature version of Unruh’s waterfall. Steinhauer filled a small vial with a cloud of very cold rubidium atoms. These atoms entered into a special state of matter wherein the speed of sound is slowed tremendously, making supersonic speeds easy to generate in this system. Steinhauer observed that on either side of this system’s event horizon, pairs of “phonons” were entangled. This is analogous to the photon pairs that are believed to form the basis of Hawking radiation in black holes. Steinhauer’s experiment was thus, by some definition, the first observation of the quantum effects of Hawking radiation.
Physicists must be cautious in relating the findings from these cultivated black holes to their natural-born cousins.
There is a diversity of materials used and mysteries explored with analogue black holes. For example, to probe what happens to light as it goes through the curved spacetime generated by an extreme gravitational pull, physicists at the University of St. Andrew created responsive optical fibers that replicate the expected deceleration of light. This system also provides another hunting ground for signs of Hawking radiation.
Different types of black holes have different properties. Those that rotate, for example, are predicted to have a positive impact on the energy of waves that come near them. This effect has now been seen in a simple bathtub set up at University of Nottingham, where water waves that came near to—but escaped—a drain received a boost in their amplitude.
Another approach to lab-built black holes focuses on the engineering of composite materials that absorb as much light as possible. These “metamaterials” can answer fundamental questions about how light behaves under extreme circumstances. More than that, the existence of these materials could influence fields far beyond black hole science. Such light-bending devices could contribute to the creation of “invisibility cloaks” or to extremely efficient solar cells, showing that even the most exotic of scientific endeavors can impact everyday life.
Analogue systems are invaluable as a means of getting a grasp on a phenomena that is ordinarily far beyond our reach. But as analogies, they have limitations, and we must be careful not to stretch them too far. The same way medical scientists can’t assume that a treatment that works for an animal will work for a human, physicists must be cautious in relating the findings from these cultivated black holes to their natural-born cousins. Indeed, the question of whether observing Hawking radiation-like effects in sonic black holes has any bearing on what happens in real ones is an open question amongst scientists and philosophers alike. The similarity of their mathematics provides hope that these models are offering a way into the study of something universally true, despite each model having its own unique features and caveats. According to philosopher of science Radin Dardashti, studying a diversity of analogue systems may actually be a good thing, because finding evidence of Hawking radiation in multiple different analogues should strengthen the case for believing it occurs in black holes.
Space-borne X-ray telescopes and underwater particle detectors are feats of engineering that offer unparalleled insights into the big questions of the universe, and their utility in the study of black holes is unquestioned. But for a scientific toolbox, diversity is key. Analogue systems, by offering a way to cultivate black holes in the lab, provide a whole new environment in which to explore, test, and observe one of the most mysterious entities in the sky.
Grace Lindsay is a neuroscientist and science writer. She is currently working on a book about how mathematics helps us understand the brain.
This essay placed first in the Black Hole Institute’s essay contest.
Additional Reading
Bourzac, K. Making a Black Hole with Metamaterials. Technologyreview.com (2009).
Castelvecchi, D. Artificial black hole creates its own version of Hawking radiation. Nature News (2016).
Dardashti, R., Thébault, K.P.Y., & Winsberg, E. Confirmation via Analogue Simulation: What Dumb Holes Could Tell Us about Gravity. The British Journal for the Philosophy of Science 68, 55-89 (2015).
Das, S. Physicists Make Artificial Black Hole Using Optical Fiber. Spectrum.ieee.org (2008).
Grush, L. How Stephen Hawking’s biggest achievement linked opposing worlds of physics. TheVerge.com (2018).
Institute of Physics. Black Holes—An essential component of our universe. Iop.com (2012).
University of Nottingham. Scientists make waves with black hole. Research.nottingham.ac.uk (2017).
Wolchover, N. What Sonic Black Holes Say About the Real Deal. Wired.com (2016).
Lead image collage credit: Vadim Sadovski / Shutterstock