Though we can see in remarkably low-light conditions, humans aren’t quite sensitive enough to see individual photons—the particles that make up all types of light. In our day-to-day lives, we are so awash with light that its particle nature is just as masked as the atomic nature of matter. We don’t feel single molecules of water, we feel the total effect of being wet; we don’t register single photons, but we can see thanks to the veritable ocean of light engulfing us.
Nevertheless, individual photons are just as real and important as individual atoms, molecules, and electrons. While it’s often hard to isolate them, single photons could be the key to new technologies, including quantum computers. Additionally, experiments on single light particles can probe many quantum aspects of existence in ways that are difficult to do with matter.
One of the most mind-bending types of single-photon research is the quantum eraser, in which pinning down the specific, measurable path of a photon “erases” some of the wave-like information in light—and vice versa. These experiments exploit the simultaneous wave-like and particle-like character of light, all the while messing with our naïve notions of time.
A staple experiment in many introductory physics classes involves shining a laser on a barrier with two small slits. The light produces a distinctive pattern on a screen, which students are tasked with measuring. The pattern is from the interference of light waves; you can see similar effects from water waves passing through barriers in a sea wall.
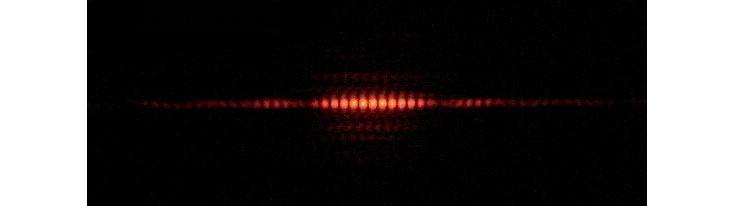
Where things get fun is if you can reduce the rate of light produced by the laser, sending photons through the barrier in small numbers. Each time you send a photon through the barrier, it hits another spot on the screen. (The position at which it lands on the screen isn’t necessarily in a direct line with either slit in the barrier, which is what you’d expect if it was following a straight path, akin to a thrown baseball.) If you draw a dot where eat photon lands, eventually you will see a pattern emerge: the very same interference pattern seen when you send trillions of photons through every fraction of a second. And that’s the kicker: If you send one photon through at a time, each one makes a discrete mark, like a particle, but all together the marks make an interference pattern, like a wave.
That combination of wave-like and particle-like aspects is essentially quantum behavior; there are no macroscopic analogs from our daily experience. Physicist Richard Feynman wrote that the weird behavior of fundamental particles is “a phenomenon which is impossible, absolutely impossible, to explain in any classical way, and which has in it the heart of quantum mechanics.”
The best way to see the quantum eraser is to couple the double-slit experiment with another fascinating quantum phenomenon: entanglement. In a typical implementation, light from a laser stimulates a certain kind of crystal, which in turn emits two photons with opposite polarization—one could oscillate left-right, while the other oscillates up-down. (You can see how this works by putting one pair of polarized sunglasses in front of another and rotating one pair. At certain angles, the light going through both lenses will fade to almost nothing, a sign that the light is passing through two filters with perpendicular orientations.)
The polarization of each photon is unknown before measurement, but because of how they’re generated, they are entangled, and measuring one can instantly affect each the other. That holds true no matter how far apart the two particles are or when the measurements are taken.
This particular quantum eraser takes two entangled photons and sends them down different paths. Photon 1 travels directly to a detector that simply counts it, while Photon 2 goes to a double-slit experiment. If things are left like that, repeating the experiment will produce the usual interference pattern for the second path. Entanglement doesn’t affect anything because we aren’t performing any measurement on either photon other than noting where they end up in the detectors.
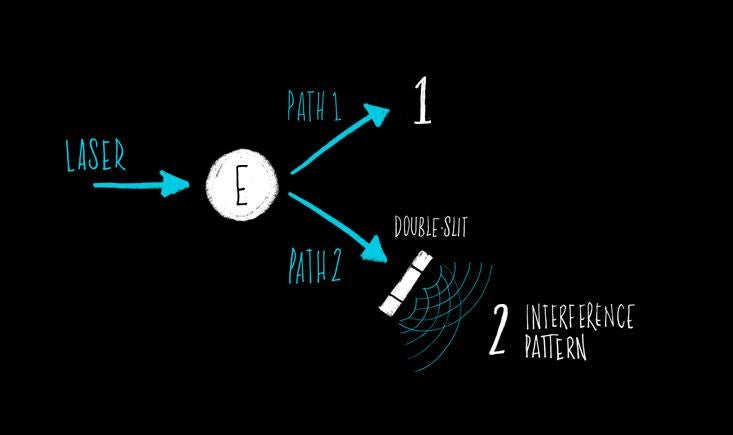
However, we can destroy the pattern by adding special filters (known as “quarter-wave plates”) to each slit. These filters ensure that Photon 2 will pass through one slit but not the other (although we can’t know which it will pass through in advance). Thus, the addition of the new filters destroys the characteristic interference pattern, “erasing” much of the wave character of the light.
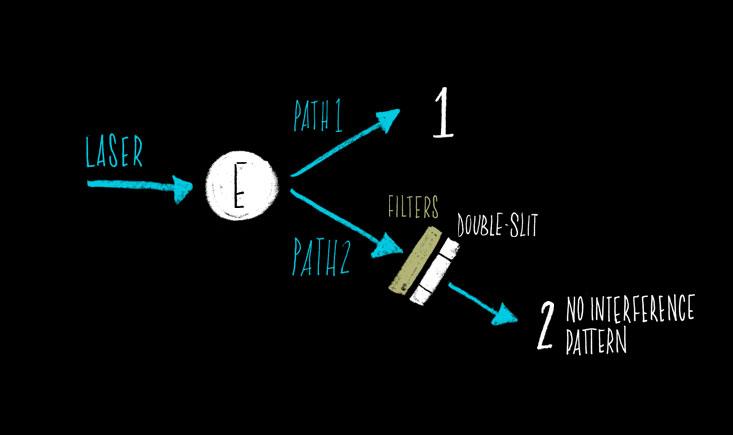
Now we restore the interference pattern by adding a simple polarizing filter aligned with the quarter-wave plates to the path of Photon 1. So Photon 1 becomes aligned with one of Photon 2’s special filters, and because the two photons are entangled, Photon 2 instantly adopts the opposite polarization, letting it go through either slit. Because Photon 2 can pass through either slit, it recovers the interference pattern and its wave-like character. At the same time, it loses the particle-like certainty that it passed through one slit or the other.
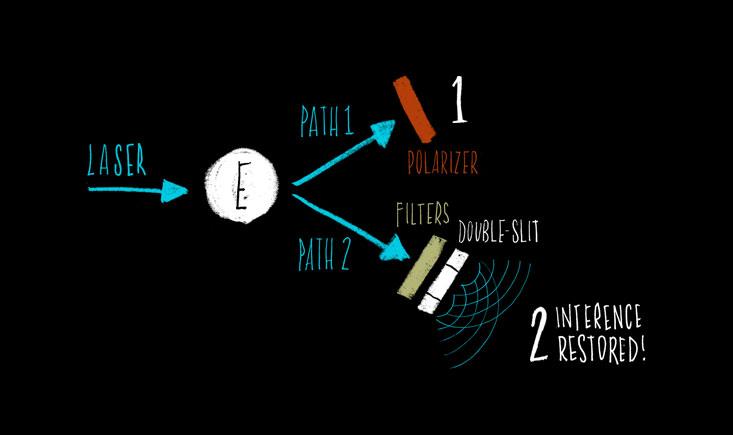
To make things even more interesting, experimenters can arrange things so that one photon travels on a much longer path than the other—even across 144 kilometers of open air between two of the Canary Islands, as one group showed last year. During transit, the researchers make a random choice whether or not to insert the polarizer in Photon 1’s very long path. Photon 2 will exhibit interference if the filter is in in Photon 1’s path, even if the polarizer is inserted after Photon 2 arrives in the detector. To put it another way: Entanglement ensures the outcome of the experiment is the same, even if it seems to be changing the past. It’s weird, but it works.
Since the quantum eraser involves individual photons, there’s no way to reduce it any further—we can’t break the experiment into smaller pieces in hopes of solving the mystery. Yet the strangeness of entanglement is part of the true character of light. The sea of photons may be what we experience, but true understanding lies in studying the smallest drop.
Matthew Francis is a physicist, science writer, public speaker, educator, and frequent wearer of jaunty hats. He’s currently writing a book on cosmology with the working title Back Roads, Dark Skies: A Cosmological Journey.