At a distance of 3.7 billion miles from the sun, on an otherwise ordinary February day in 1990, the Voyager 1 probe turned to point its camera platform away from its headlong rush into the cosmic void. A narrow-angle optic switched on and snapped a sequence of brief shots.
One of the sensor’s tiny pixels, spanning about the length of a dozen bacteria lined up end to end, registered an increased charge. There, among the sun’s lens flare, diffracted light, and electronic noise, sat an extra point of light. It was the smallest of small blips, an inconsequential mote in the emptiness of space, and it had a pale blue hue.
This mote was us, our world, Earth—a shockingly abrupt summation of 4 billion years of complex and chaotic history. The image taken that day was the ultimate selfless selfie. It provided an existential perspective that is unique among the species on our planet: Only we humans have seen ourselves from billions of miles away. Carl Sagan would later immortalize the image with the title, the “Pale Blue Dot.”
The Pale Blue Dot was the latest in a long history of association of the color blue with our home in the universe, and with life itself. Two decades before Voyager, as Apollo 8 circled the moon, astronauts recorded the now-famous “Earthrise”—a stunning color photograph of a bright blue-white hemisphere suspended above a gray lunar landscape. Four years later, the Apollo 17 mission captured a beguiling whole-Earth image that became “The Blue Marble.”
Powerful thermal gradients drive winds to thousands of miles an hour while a hot rain of glassy silicates beats its way through the maelstrom.
These space-age portraits drive home the fact that we evolved on a blue planet. Some people, like Wallace Nichols, the scientist and author of Blue Mind, have suggested that we have a primal, near irrational, desire for water and its blue oceans and lakes. This thesis is supported by a recent global survey suggesting that blue is our favorite color. In the United Kingdom alone, a world-beating 33 percent of respondents ranked blue above anything else, with red a runner-up at about 15 percent.
Blue has made it into our flags and emblems, too. NASA, the European Space Agency (ESA), the Search for Extraterrestrial Intelligence (SETI), and the Planetary Society are represented by blue backdrops, or abstract blue Earths, or blue symbols. The One Flag in Space initiative advocates a Blue Marble flag, in which a photo of the Earth is shown on a blue backdrop. Just this May, a Swedish design student named Oskar Pernefeldt proposed an International Flag of the Planet Earth that has attracted wide media coverage, consisting of seven interlocking circles on a vivid blue background.
Blue has become the default color of a living planet, a cosmic oasis, a life-harboring, fragile, and tiny jewel sitting in space. But there’s a catch: The very science and technology that gave us the Pale Blue Dot in the first place is undermining what we thought blue meant.
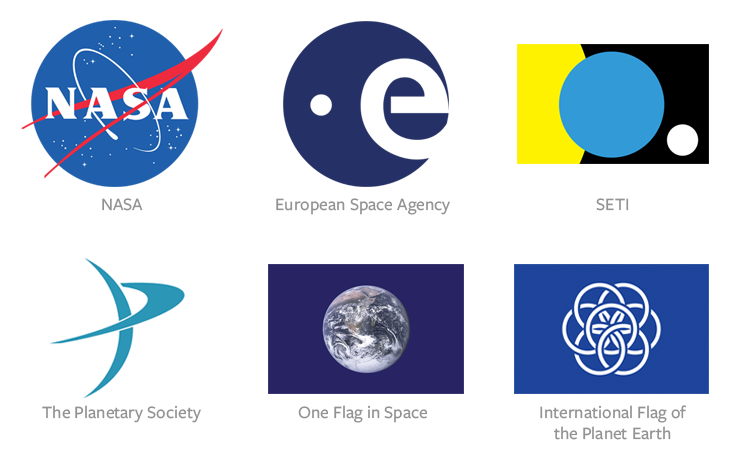
Around a star called Henry Draper 189733, some 63 light-years from Earth, is a giant planet just a little larger than Jupiter. This gas-cloaked world takes about two Earth days to complete an orbit. It lives incredibly close to its stellar parent. As a result, the upper planetary atmosphere is heated to over 1,300 degrees Fahrenheit (or over 700 degrees Celsius). Powerful thermal gradients drive winds to thousands of miles an hour while a hot rain of glassy silicates beats its way through the maelstrom. It is, in other words, a truly beastly place.
By stretching current astronomical instruments to their limit, scientists have been able to make a first, crude measurement of how this planet reflects starlight, and to divine its overall color. Which is a rather serene, deep azure blue, not unlike that of Earth’s skies on a summer’s day.
Astronomers’ best guess is that this world has a moderate cloud deck of condensed, but still scorching, minerals—with a clear atmosphere above it. The clouds reflect most light, but molecular hydrogen in the higher atmosphere preferentially diverts and polarizes blue wavelengths. That upper atmosphere may also contain sodium atoms that absorb red wavelengths. The upshot? The planet is another blue marble, but very unlike Earth.
We don’t even have to look as far away as HD 189733b to find blue planets. In fact, Earth isn’t the only Pale Blue Dot in its own solar system. If you take a look at Voyager’s full family portrait of planets, you’ll notice that both Uranus and Neptune could, at a glance, be called pale blue (actually, Neptune is more of a strong azure). In neither case is it water oceans or Earth-like atmospheres producing our favorite color.
The dominant blue in these hulking ice giants is caused by trace amounts of methane lurking in their upper atmospheres. Methane molecules absorb light in various bands of the red and infrared spectrum, leaving the blue reflected light alone. The difference in blue tones between these planets is, however, still a bit of a mystery. Neptune’s vigorous atmospheric upheavals, which loft compounds like ammonia and water ice up from interior layers, may be responsible for its deeper shade of blue. Its stratosphere may also contain a greater number of hydrocarbons.
The bottom line is that blue may correspond to sterile, inhospitable places just as much as it may reflect a planet ripe for being inhabited. The Pale Blue Dot turns out to be a lousy signpost for life.
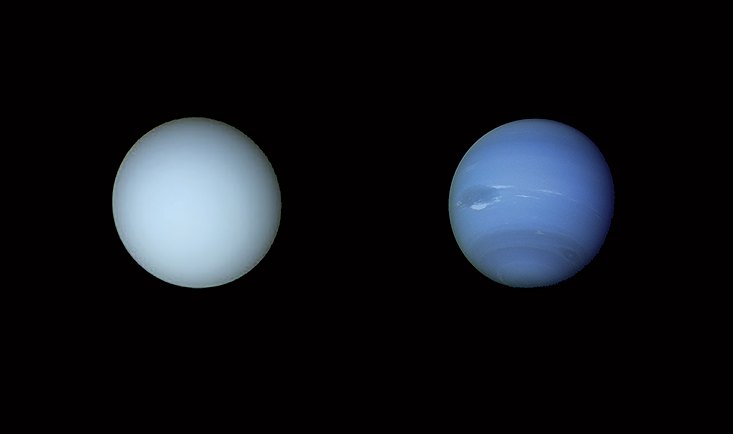
Suppose that, instead of obsessing over colors that indicate the right conditions for life, we look for the colors of life itself? On modern Earth, the dominant light-harvesting mechanisms for life employ a set of pigments we call chlorophylls. These molecules preferentially absorb light in the blue and red, reflecting back greenish tones. As a result, near the surface of Earth’s oceans, microscopic photosynthetic organisms can create vast green blushes, mixed with the scattered blue light of pure water. On dry land, plant life can also produce great swathes of green, blending with the inorganic hues of the planet.
If we could see these signatures in Earthshine—the summed up, one-shot, reflected light of the whole planet—we might be able to tease out the signs of life, and then look for those same signs in distant planets. But evaluating Earthshine is difficult because we seldom have the right spectrographic instruments and telescopes sitting off world. So astronomers have resorted to ingenious methods that measure Earthshine as it reflects from the night side of the moon. It’s a technique that was used from the 1920s to the 1960s to evaluate Earth’s reflectivity, or albedo, but then largely forgotten.
One modern approach involves lining up the slit of a telescope’s spectrograph across different parts of the lunar disk, and undertaking a hair-raising set of geometric calculations to figure out what part of Earth’s dayside is illuminating the moon—then considering the complex effects of lunar reflection and scattering. This is not an easy experiment, with photons being further absorbed and scattered as they travel back and forth through Earth’s atmosphere. But it can be done, and some of the results suggest that finding the green tint of photosynthetic life from bulk Earthshine is disappointingly hard. This pigmentation seems to contribute little to the total spectrum, although that could depend acutely on exactly which oceans and continents are bouncing light to the moon at a given moment.
The dim reddish spectrum of starlight could encourage the natural selection of organisms that capture all parts of the visible spectrum: Black wins.
What’s more, green may not be the right color to look for. Even on Earth, there are a variety of different pigments that can be involved in photosynthesis. So-called “accessory” pigments serve as intermediaries that pass on absorbed photon energy to chlorophylls for final processing. Carotenoid accessory pigments can appear red, orange, or yellow. Microbial species that use both carotenoids and chlorophylls can tweak the mix depending on their environment—cranking up the yellows and oranges in conditions of harsh sunlight or high temperatures, or switching down to browns and greens in cold or wintery conditions. Another class of accessory pigments called phycobilins can be blue or red.
The colors of life can also change over geological time scales. In 2006 the astronomer Bill Sparks and the microbial geneticist Shil DasSarma suggested that a pigment called retinal—a form of vitamin A—might have been widely deployed by ancient photosynthesizing organisms. Retinal is simpler in molecular structure than chlorophyll, and certain species of tough halophilic (salt-loving) archaea use it for photosynthesis today—species that also hold up well against intense UV radiation. Retinal absorbs green light, with maximum absorption at a wavelength of around 568 nanometers, close to the maximum in the energy density of the sun’s spectrum. The result is that retinal membranes used for photosynthesis also reflect nicely in the red and blue, producing a rich purple color. In other words, 3 billion years ago, on an Earth harboring microbial life in a largely oxygen-free atmosphere, there is a chance that the planetary surface was covered in purple. Should we be scanning the heavens for pale purple dots?
Or maybe black is the right color. The overwhelming majority of stars in the Milky Way are smaller, fainter, and colder than the sun. My colleague Nancy Kiang, at NASA’s Goddard Institute for Space Studies in New York City, has argued that, on these worlds, the dim reddish spectrum of starlight could encourage the natural selection of organisms that capture all parts of the visible spectrum: Black wins. The trouble, of course, is that black is hard to find. A distant astronomer trying to detect and decipher a planet’s biological systems through color might be out of luck.
To complicate things even further, we don’t yet have any instrument sensitive enough to peer at the reflected colors due to life on Earth-analog exoplanets (though we are at least gaining insight into what it would take to do this: huge light-gathering telescopes and optical trickery to remove glaring starlight). Yes, life can be colorful, but looking for it that way is a tricky proposition.
The truth is that colors are a cosmically parochial idea. The electromagnetic spectrum is not inherently divisible into neat pieces, except by limited sensory facilities. Light at different wavelengths is entwined with a vast array of physical processes, from the probabilistic hop and skip of electrons between atomic energy levels, to scattering and diffraction by matter’s electromagnetic fields. Wildly disparate processes can produce or consume photons of identical energy, generating almost indistinguishable gross features such as color. And sometimes a single underlying physical process can make utterly dissimilar objects appear the same.
Into this morass steps the phenomenon of life. Physics and chemistry are important for gross planetary color, but not necessarily biology. Naïvely equating the color blue with habitable environments has some serious flaws.
But that doesn’t mean that color is useless to the cosmic life hunter. A biosphere clearly brings new structure to color, modifying a planetary spectrum in ways that could be pronounced and specific.
A classic example is the famous “vegetation red-edge”—a sharp enhancement of transmission and reflectivity of photons by the cells of plant life here on Earth. At wavelengths longer than about 0.7 micrometers, plants start reflecting approximately 50 percent of the infrared light hitting them—more than a tenfold step up in reflected brightness compared to the shorter wavelengths of visible red.
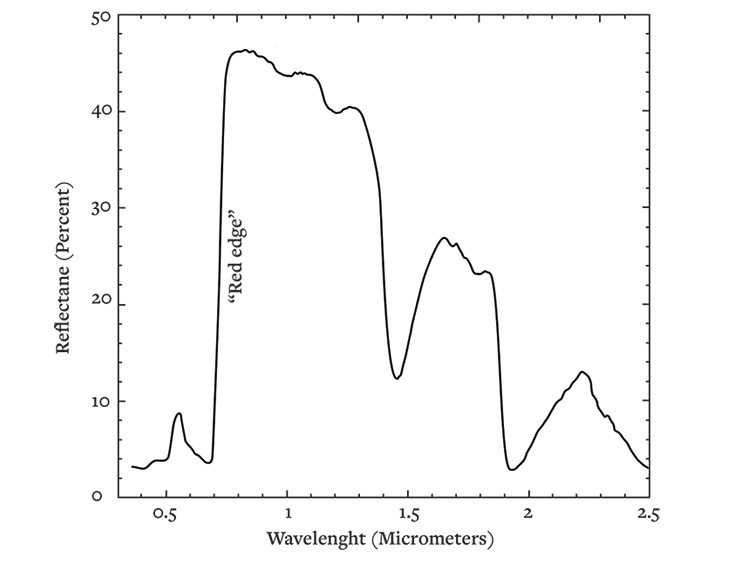
The origins of the effect are still incompletely understood. It seems that the internal optics of plant cells are largely responsible. At cell walls, water-to-air boundaries result in mirror-like critical angle reflections. Infrared photons bounce between these boundaries, then get efficiently ejected. What plant life gains from this is not entirely clear. It’s possible that rejecting near-infrared radiation helps with temperature regulation.
Whatever its origin, the red edge is hugely useful to us, enabling remote sensing satellites to map out Earth’s jungles, grasslands, and crops. However, since a randomly illuminated crescent of a world like the Earth is on average 70 percent ocean, with an average of 70 percent cloud cover, red-edge optics can amount to less than a 10 percent feature in the total planetary spectrum. Get lucky though, and the signal can be much stronger.
Life also brings strong temporal structure to color. Seasons impact planetary habitats, and organisms can be triggered to bloom and die. For example, in 2008, iron-rich ash from the Kasatochi Volcano in the Northeastern Pacific caused a huge green growth of plankton, visible from space across 2 million square kilometers of ocean, in mere days. Changing colors on exoplanets could potentially provide a signature of life, even if we did not understand what the colors themselves meant.
Color, then, will no doubt remain an important part of the astrobiologist’s toolkit. It just may not be as simple as “spot the blue dot.” In this sense we should see the Voyager image as one of great hope. It was merely the opening clause in a dialogue with the universe. It was the day we finally got to watch our deceptively simple colors flying among an armada of new worlds.
Caleb Scharf is an astrophysicist and the Director of Astrobiology at Columbia University in New York. His latest book is The Copernicus Complex: Our Cosmic Significance in a Universe of Planets and Probabilities.
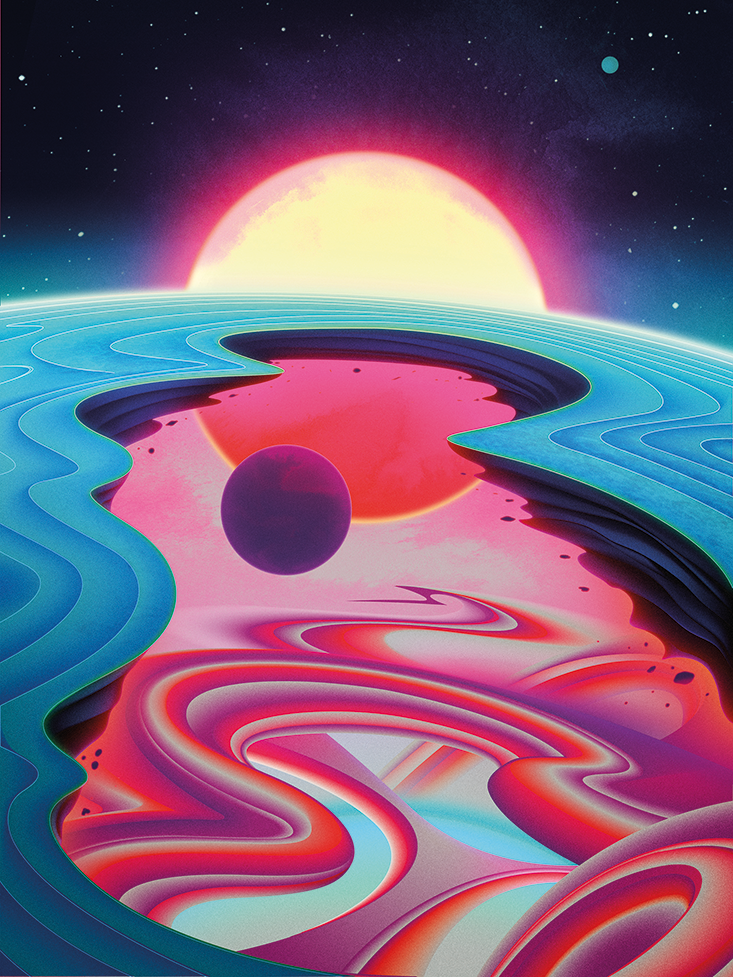