All we are is dust in the wind, man. The same goes for the planets and asteroids and comets. Starting from our dusty beginnings, gravity and a mess of other forces conspired to build our solar system. There’s a venerable tradition of trying to figure out what that grand and hectic process must have looked like. Today, with the aid of sophisticated simulations, scientists can meticulously tinker with models that might show us how the solar system got to be the way it is now. In a new Nature Astronomy paper, we take a step forward by building on some of the most compelling ideas to date.
This has long been an alluring challenge. The German bigwig philosopher Immanuel Kant and one of France’s most important scientific theorists, Pierre Laplace, both 18th-century thinkers, thought it all started from a disk of dust and gas going round the young sun. Later, about a century ago, Thomas Chamberlain and Forest Moulton proposed a different idea, that the planets formed out of city- to county-sized rocky bodies called planetesimals. It turns out that both ideas are basically correct. Anyone today can spend an hour looking through NASA images from telescopes revealing disks around young stars that astronomers suspect are forming planets as you read. Asteroids and comets are evidence that rocky and icy planetesimals formed throughout the solar system.
A Frankensteinian monster of a model.
We propose that the planets formed from three separate rings of planetesimals within a gaseous disk around the sun. This model also makes sense of planetary disks around other stars, connecting them with the orbits of our solar system’s planets and asteroids, as well as chemical measurements of meteorites. Why rings of planetesimals? This concept starts to make sense if you squint at the solar system from a large distance and imagine spreading out the mass that makes up the planets: Almost all of the rocky material concentrates between the orbits of Venus and Earth with very little mass closer to the sun or in the asteroid belt, while a little farther from the sun, Jupiter and Saturn make up a huge amount of mass that tapers off to the outer solar system. But what determines the properties of these rings?
Planetesimals can coalesce spontaneously whenever there’s enough dust clumped within a specific location within a gas-dominated disk. Dust grains grow in dust-grain collisions, and when they reach roughly a millimeter in size, they start to experience drag as if biking against the wind. This causes large dust grains to drift inward, toward the sun. Modeling has shown that there exist dust “traps” within the disk, associated with bumps in the local gas pressure.
Scientists often see such ringed traps in images of planet-forming disks. You can see some in the image above, taken with the ALMA (Atacama Large Millimeter Array) telescope in Chile. Dust traps can be associated with specific important molecules evaporating and condensing. In our model, these end up being silicate rocks, water, and carbon monoxide—they’re linked to our three strongest traps. The condensation temperatures of these elements span from 30 degrees above absolute zero (for carbon monoxide) to 1500 degrees (for silicates). Each corresponds to a given orbital distance from the star. Drifting dust piles up at each of these locations within the disk and produces a ring of planetesimals. These three rings are, in our model, the building blocks of the planets.
You can directly connect each ring with a specific region of our solar system. That’s pretty neat. In our simulations, the inner ring contains two to three times as much mass as Earth in rocky planetesimals. The two most massive terrestrial planets, Venus and Earth, formed within this ring; Mars and Mercury were scattered out of the ring, their growth stunted. Mars grew mostly from material in the ring’s outer parts, which nicely explains the chemical difference between Mars and the Earth (Earth is more similar in composition to one group of meteorites and Mars to another.)
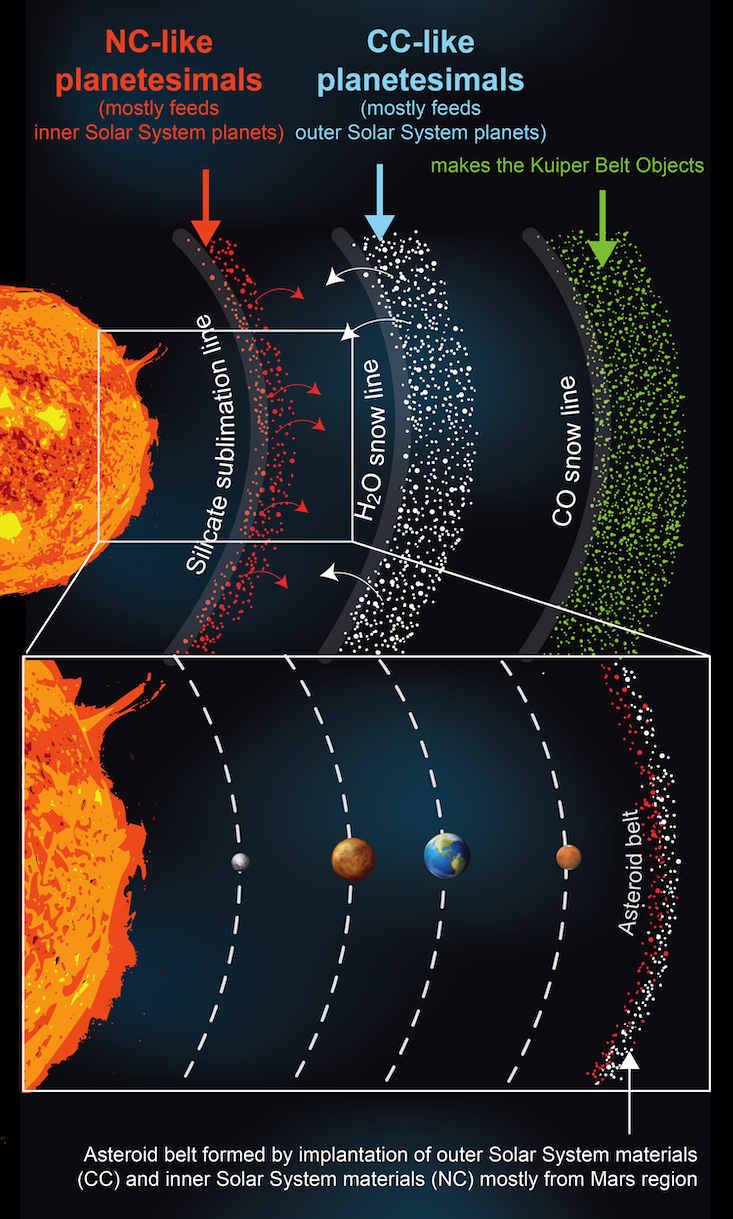
The middle ring is, in our simulations, the most massive, with 50 to 100 Earth masses in planetesimals. Massive planets, of 10 to 20 Earth masses, grow quickly within the ring by colliding with dust and other planetesimals. These, in virtue of their gravity, capture gas from the disk and grow into Jupiter and Saturn. The ice giants Uranus and Neptune also formed within the outskirts of this ring, but their slower growth prevented them from capturing more gas.
And the asteroid belt? That lies between the inner and middle rings. In our simulations, it can be thought of as a cosmic “refugee camp.” It contains objects that formed across the solar system but perhaps not within the belt, and births few planetesimals and sometimes none at all. This matches the observed orbital distribution as well as the chemical gradients across the belt that are inferred from meteorites linked with different asteroid types. The present-day belt only contains a total of less than 0.05 percent of an Earth mass, consisting of planetesimals scattered outward from the inner ring during the growth of the rocky planets, and planetesimals scattered inward from the middle ring during the growth of the gas- and ice giants.
The outer ring of planetesimals corresponds to the present-day Kuiper belt, the population of small icy bodies beyond the orbit of Neptune. While our simulations typically produce 20 to 30 Earth masses in planetesimals—two orders of magnitude more than the present-day Kuiper belt—it conveniently matches the amount of mass needed in an outer belt to explain the giant planets’ current orbits.
So, our three-ring model reproduces what you could call our solar system’s orbital architecture. It would be impressive if these same processes could explain the diversity of other exoplanet systems. And we think they can.
Planet-forming disks around other stars are ubiquitous, with a spectrum of different properties, but planetesimal rings should form systematically. Close-in super-Earth, or sub-Neptune planets, found around roughly 30 percent of all stars may form from planetesimals within inner or middle rings. If the planets reach Mars’ size, about 10 percent of Earth’s mass, before the gas disappears from the disk, which can take a few million years, then they launch spiral density waves. These cause the planets’ orbits to shrink, or migrate, toward the central star, an outcome our solar system likely avoided because our rocky planets grew too slowly. Gas giant planets should most naturally form from the middle rings, and, indeed, most giant exoplanets are found on orbits wider than super-Earths’ but still closer to Earth’s orbit than Jupiter’s (perhaps due to a modest degree of migration).
Moulton, one of the proponents of the old planetesimal hypothesis, once stressed how utterly distinct this idea was from the dusty-disk one Laplace had. “The gap,” he wrote in a 1928 issue of Science, “between these different genera of intellectual constructions is as profound as that between different genera of living organisms, and as difficult to bridge.” He was, as you might guess, pretty wrong about that. Our three-ring model bridges or synthesizes those ideas, along with several others, to make what Moulton—sticking with his analogy to life—might have called a complicated chimera. A Frankensteinian monster of a model. As long as it’s useful, that’s fine with us.
Sean Raymond is an American astrophysicist working at the Bordeaux Astrophysical Laboratory in France. He also writes a blog at the interface of science and fiction (planetplanet.net), and recently published a book of astronomy poems.
Andre Izidoro is a postdoctoral scholar in the Department of Earth, Environmental and Planetary Science at Rice University.
Rajdeep Dasgupta is a cosmochemist in the Department of Earth, Environmental and Planetary Science at Rice University. He leads the Experimental Petrology Team and the CLEVER-Planets project.
Lead image: The ALMA telescope, in Chile, sensitive to millimeter-sized dust, took these images of planet-forming disks.ALMA (ESO/NAOJ/NRAO), S. Andrews et al.; NRAO/AUI/NSF, S. Dagnello