Ever since the dawn of the space age, a quixotic subculture of physicists, engineers, and science-fiction writers have devoted their lunch hours and weekends to drawing up plans for starships, propelled by the imperative for humans to crawl out of our Earthly cradle. For most of that time, they focused on the physics. Can we really fly to the stars? Many initially didn’t think so, but now we know it’s possible. Today, the question is: Will we?
Truth is, we already are flying to the stars, without really meaning to. The twin Voyager space probes launched in 1977 have endured long past their original goal of touring the outer planets and have reached the boundaries of the sun’s realm. Voyager 1 is 124 astronomical units (AU) away from the sun—that is, 124 times farther out than Earth—and clocking 3.6 AU per year. Whether it has already exited the solar system depends on your definition of “solar system,” but it is certainly way beyond the planets. Its instruments have witnessed the energetic particles and magnetic fields of the sun give way to those of interstellar space—finding, among other things, what Ralph McNutt, a Voyager team member and planetary scientist, describes as “weird plasma structures” begging to be explored. The mysteries encountered by the Voyagers compel scientists to embark on follow-up missions that venture even deeper into the cosmic woods—out to 200 AU and beyond. But what kind of spacecraft can get us there?
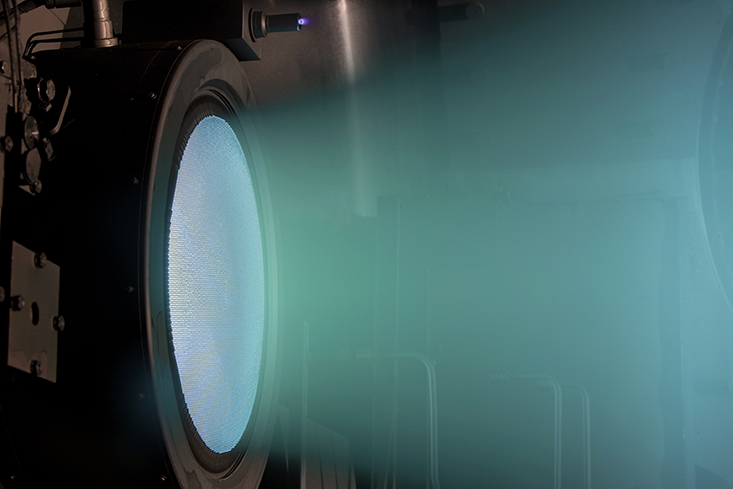
Going Small: Ion Drives
NASA’s Dawn probe to the asteroid belt has demonstrated one leading propulsion system: the ion drive. An ion drive is like a gun that fires atoms rather than bullets; the ship moves forward on the recoil. The system includes a tank of propellant, typically xenon, and a power source, such as solar panels or plutonium batteries. The engine first strips propellant atoms of their outermost electrons, giving them a positive electric charge. Then, on the principle that opposites attract, a negatively charged grid draws the atoms toward the back of the ship. They overshoot the grid and stream off into space at speeds 10 times faster than chemical rocket exhaust (and 100 times faster than a bullet). For a post-Voyager probe, ion engines would fire for 15 years or so and hurl the craft to several times the Voyagers’ speed, so that it could reach a couple of hundred AU before the people who built it died.
Star flight enthusiasts are also pondering ion drives for a truly interstellar mission, aiming for Alpha Centauri, the nearest star system some 300,000 AU away. Icarus Interstellar, a nonprofit foundation with a mission to achieve interstellar travel by the end of the century, has dreamed up Project Tin Tin—a tiny probe weighing less than 10 kilograms, equipped with a miniaturized high-performance ion drive. The trip would still take tens of thousands of years, but the group sees Tin Tin less as a realistic science mission than as a technology demonstration.
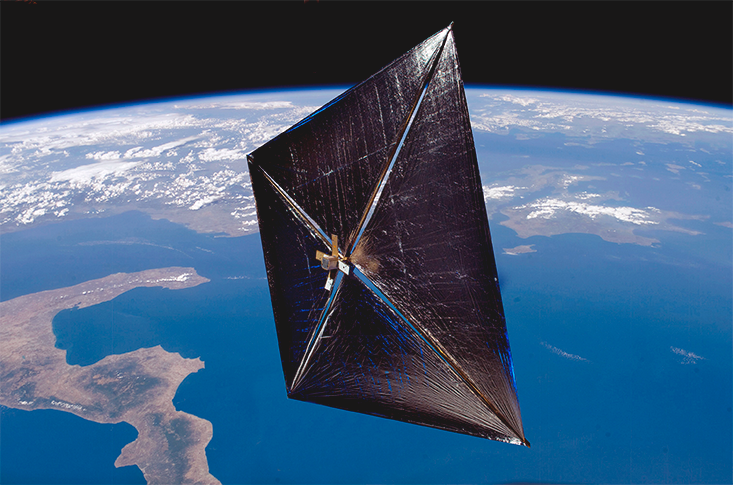
Going Light: Solar Sails
A solar sail, such as the one used by the Japanese IKAROS probe to Venus, does away with propellant and engines altogether. It exploits the physics of light. Like anything else in motion, a light wave has momentum and pushes on whatever surface it strikes. The force is feeble, but becomes noticeable if you have a large enough surface, a low mass, and a lot of time. Sunlight can accelerate a large sheet of lightweight material, such as Kapton, to an impressive speed. To reach the velocity needed to escape the solar system, the craft would first swoop toward the sun, as close as it dared—inside the orbit of Mercury—to fill its sails with lusty sunlight.
Such sail craft could conceivably make the crossing to Alpha Centauri in a thousand years. Sails are limited in speed by how close they can get to the sun, which, in turn, is limited by the sail material’s durability. Gregory Matloff, a City University of New York professor and longtime interstellar travel proponent, says the most promising potential material is graphene—ultrathin layers of carbon graphite.
Like anything else in motion, a light wave has momentum and pushes on whatever surface it strikes.
A laser or microwave beam could provide an even more muscular push. In the mid-1980s, the doyen of interstellar travel, Robert Forward, suggested piggybacking on an idea popular at the time: solar-power satellites, which would collect solar energy in orbit and beam it down to Earth by means of microwaves. Before commencing operation, an orbital power station could pivot and beam its power up rather than down. A 10-gigawatt station could accelerate an ultralight sail—a mere 16 grams—to one-fifth the speed of light within a week. Two decades later, we’d start seeing live video from Alpha Centauri.
This “Starwisp” scheme has its dubious features—it would require an enormous lens, and the sail is so fragile that the beam would be as likely to fry it as to push it—but it showed that we could reach the stars within a human lifetime.
Going Big: Nuclear Rockets
Sails may be able to whisk tiny probes to the stars, but they can’t handle a human mission; you’d need a microwave beam consuming thousands of times more power than the entire world currently generates. The best-developed scheme for human space travel is nuclear pulse propulsion, which the government-funded Project Orion worked on during the 1950s and ’60s.
When you first hear about it, the scheme sounds unhinged. Load your starship with 300,000 nuclear bombs, detonate one every three seconds, and ride the blast waves. Though extreme, it works on the same basic principle as any other rocket—namely, recoil. Instead of shooting atoms out the back of the rocket, the nuclear-pulse system shoots blobs of plasma, such as fireballs of tungsten.
You pack a plug of tungsten along with a nuclear weapon into a metal capsule, fire the capsule out the back of the ship, and set it off a short distance away. In the vacuum of space, the explosion does less damage than you might expect. Vaporized tungsten hurtles toward the ship, rebounds off a thick metal plate at the ship’s rear, and shoots into space, while the ship recoils, thereby moving forward. Giant shock absorbers lessen the jolt on the crew quarters. Passengers playing 3-D chess, or doing whatever else interstellar passengers do, would feel rhythmic thuds like kids jumping rope in the apartment upstairs.
Load your starship with 300,000 nuclear bombs, detonate one every three seconds, and ride the blast waves.
The ship might reach a tenth the speed of light. If for some reason—solar explosion, alien invasion—we really had to get off the planet fast and we didn’t care about nuking the launch pad, this would be the way to go. We already have everything we need for it. “Today the closest technology we have would be nuclear pulse,” Matloff says. If anything, most people would be happy to load up all our nukes on a ship and be rid of them.
Ideally, the bomb blasts would be replaced with controlled nuclear fusion reactions. That was the approach suggested by Project Daedalus, a ’70s-era effort to design a fully equipped robotic interstellar vessel. The biggest problem was that for every ton of payload, the ship would have to carry 100 tons of fuel. Such a behemoth would be the size of a battleship, with a length of 200 meters and a mass of 50,000 tons.
“It was just a huge, monstrous machine,” says Kelvin Long, an English aerospace engineer and co-founder of Project Icarus, a modern effort to update the design. “But what’s happened since then, of course, is microelectronics, miniaturization of technology, nanotechnology. All these developments have led to a rethinking. Do you really need these massive structures?” He says Project Icarus plans to unveil the new design in London this October.
Interstellar designers have come up with all sorts of ways to shrink the fuel tank. For instance, the ship could use electric or magnetic fields to scoop up hydrogen gas from interstellar space. The hydrogen would then be fed into a fusion reactor. The faster the ship were to go, the faster it would scoop—a virtuous cycle that, if maintained, would propel the ship to nearly the speed of light. Unfortunately, the scooping system would also produce drag forces, slowing the ship, and the headwind of particles would cook the crew with radiation. Also, pure-hydrogen fusion is inefficient. A fusion-powered ship probably couldn’t avoid hauling some fuel from Earth.
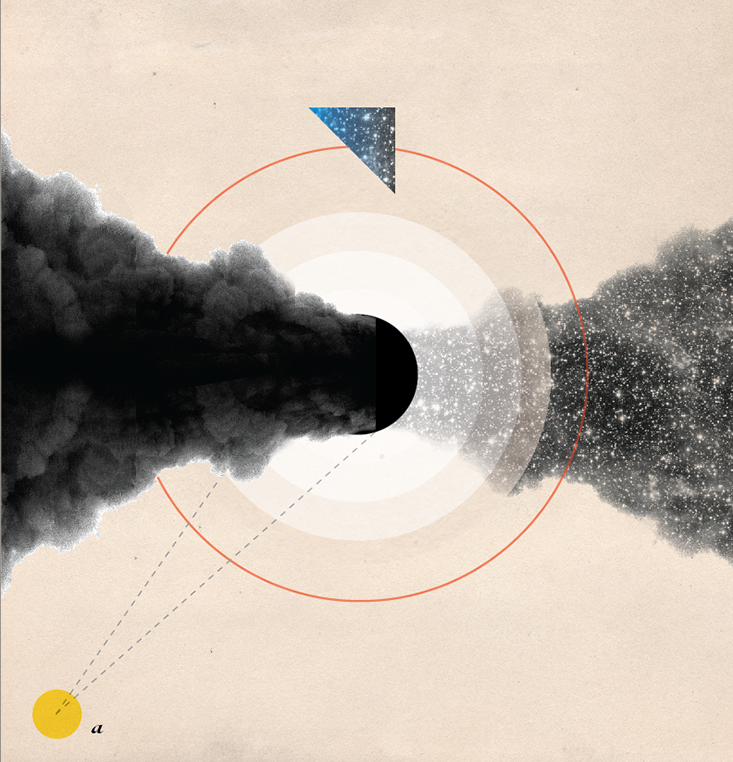
Going Dark: Scavenging Exotic Matter
Instead of scavenging hydrogen gas, Jia Liu, a physics graduate student at New York University, has proposed foraging for dark matter, the invisible exotic material that astronomers think makes up the bulk of the galaxy. Particle physicists hypothesize that dark matter consists of a type of particle called the neutralino, which has a useful property: When two neutralinos collide, they annihilate each other in a blaze of gamma rays. Such reactions could drive a ship forward. Like the hydrogen scooper, a dark-matter ship could approach the speed of light. The problem, though, is that dark matter is dark—meaning it doesn’t respond to electromagnetic forces. Physicists know of no way to collect it, let alone channel it to produce rocket thrust.
If engineers somehow overcame these problems and built a near-light-speed ship, not just Alpha Centauri but the entire galaxy would come within range. In the 1960s astronomer Carl Sagan calculated that, if you could attain a modest rate of acceleration—about the same rate a sports car uses—and maintain it long enough, you’d get so close to the speed of light that you’d cross the galaxy in just a couple of decades of shipboard time. As a bonus, that rate would provide a comfortable level of artificial gravity.
On the downside, hundreds of thousands of years would pass on Earth in the meantime. By the time you got back, your entire civilization might have gone ape. From one perspective, though, this is a good thing. The tricks relativity plays with time would solve the eternal problem of too-slow computers. If you want to do some eons-long calculation, go off and explore some distant star system and the result will be ready for you when you return. The starship crews of the future may not be voyaging for survival, glory, or conquest. They may be solving puzzles.
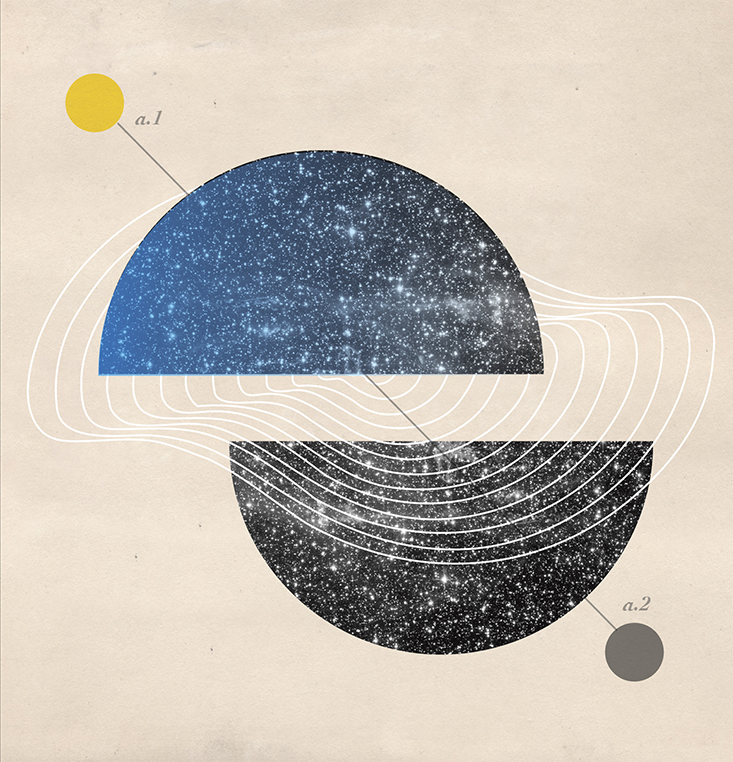
Going Warp: Bending Time and Space
With a ship moving at a tenth the speed of light, humans could migrate to the nearest stars within a lifetime, but crossing the galaxy would remain a journey of a million years, and each star system would still be mostly isolated. To create a galactic version of the global village, bound together by planes and phones, you’d need to travel faster than light.
Contrary to popular belief, Einstein’s theory of relativity does not rule that out completely. According to the theory, space and time are elastic; what we perceive as the force of gravity is in fact the warping of space and time. In principle, you could warp space so severely that you’d shorten the distance you want to cross, like folding a rug to bring the two sides closer together. If so, you could cross any distance instantaneously. You wouldn’t even notice the acceleration, because the field would zero out g-forces inside the ship. The view from the ship windows would be stunning. Stars would change in color and shift toward the axis of motion.
You could warp space so severely that you’d shorten the distance you want to cross, like folding a rug to bring the two sides closer together.
It seems almost mean-spirited to point out how far beyond our current technology this idea is. Warp drive would require a type of material that exerts a gravitational push rather than a gravitational pull. Such material contains a negative amount of energy—literally less than nothing, as if you had a mass of –50 kilograms. Physicists, inventive types that they are, have imagined ways to create such energy, but even they throw up their hands at the amount of negative energy a starship would need: a few stars’ worth. What is more, the ship would be impossible to steer, since control signals, which are restricted to the speed of light, wouldn’t be fast enough to get from the ship’s bridge to the propulsion system located on the vessel’s perimeter. (Equipment within the ship, however, would function just fine.)
When it comes to starships, it’s best not to get hung up on details. By the time humanity gets to the point it might actually build one, our very notions of travel may well have changed. “Do we need to send full humans?” asks Long. “Maybe we just need to send embryos, or maybe in the future, you could completely download yourself into a computer, and you can remanufacture yourself at the other end through something similar to 3-D printing.” Today, a starship seems like the height of futuristic thinking. Future generations might find it quaint.
George Musser is a writer on physics and cosmology and author of The Complete Idiot’s Guide To String Theory (Alpha, 2008). He was a senior editor at Scientific American for 14 years and has won honors such as the American Institute of Physics Science Writing Award.