If you’ve never really noticed the wide range of colors that can adorn the domestic cat, you might want to spend some time skimming through the official color charts of the Cat Fanciers Association website. According to the association, which claims to maintain the largest registry of pedigreed cats, cats can come in seal lynx and mackerel tabby, chinchilla silver and cream smoke, blue-patched and blue point. There are mitted cats and van cats, as well as more obvious cats that you might actually be able to picture in your mind, like “green-eyed white.” (Here is a very detailed poster showing much of the complexity in distinguishing breeds.)
This Crayola box of fur is enabled by just a handful of genes, leveraged by a long history of human breeders obsessed with getting the rarest or most beautiful or most striking combinations. But in that world of cat possibility, perhaps the strangest and most interesting of all is the common calico cat. All calicos (and all tortoiseshells) have blotches of black and orange fur. They are also almost always female. The reason why has to do with a genetic phenomenon that gets down to the very roots of what it means to be female.
It was only a bit more than a hundred years ago that biologists realized that male and female mammals actually were different in the structure of their chromosomes, the packages that house their DNA, with females having two X chromosomes and males having an X and a Y. But the strange thing was that the human body worked perfectly well either way. Normally, variation in the number of chromosomes causes significant genetic disease; for example, people with extra copy of chromosome 21 have Down syndrome, which usually comes with developmental and cognitive problems. Since females have twice as many X-chromosome genes, they should have twice the appropriate amount of proteins coded by those genes, leading to a lot of biological errors. Yet they are healthy. In women, there evidently was some trick that offset the doubling of the X chromosome, but it was a mystery.
In 1959 a researcher noticed that in cells from female mammals, one of the two X chromosomes looked funny during the resting period of the cell cycle; it shriveled up into a blob and clung to the outer edge of the cell nucleus. For genes to be transcribed, a chromosome must loosen up its structure so that its DNA can be physically accessed by the transcription machinery. These chromosomes, one inside each cell, looked like they couldn’t possibly be doing anything.
Two years later, the British geneticist Mary Lyon had the idea that what he was looking at was also the way that female cells compensate for having twice as much X. She proposed that each cell randomly and permanently deactivates one of its X chromosomes, disabling one of them and preventing the cell from being swamped with protein. She was right, and the discovery of X-inactivation (now sometimes called lyonization) was the first description of an epigenetic phenomenon—the realization that gene activity can be permanently changed by something other than heritable changes to DNA.
Each calico is both an orange cat and a black cat, and there will never again be one like her. “Identical” twins don’t even have the same patches, because chance dictates which X-chromosomes are inactivated.
Since then, more details have been worked out: When an egg (which has just one X chromosome) first meets and unites with a sperm cell that has an X chromosome (one half of sperm cells have Xs and lead to girls; half have Ys and lead to boys), both X chromosomes are active. The fertilized egg grows and divides into a ball of hundreds of cells, still with both X’s active. Then after a little more than a week, a gene called XIST (X-inactive specific transcript) springs into action, producing an RNA that tightly binds one of the two chromosomes, wrapping it up so snugly as to prevent almost all its genes from being transcribed. The silenced X chromosome will stay that way throughout the life of the cell. Each time it divides, its daughter cells will maintain the same pattern of inactivation.
The system works the same in all female placental mammals. (Marsupials have their own weird system for doing the same thing.) But in calico and tortoiseshell cats, it becomes gloriously visible.
On the X chromosome of cats is a skin- and fur-color gene that has two variations (alleles) that dictate either orange fur or black fur. If a female cat inherits one X chromosome with the black allele and one with the orange version, each cell will have both versions, but X-inactivation means that some of her skin cells will code for orange and some for black. The inactivation happens very early in development, when the cat-in-the-making is still just a ball of cells, and the particular nature of skin tissue is that cells and their progeny stay close together. One of those primordial skin progenitor cells that happens to have an active orange allele will give rise to a cohesive blob of millions of cells in the fully developed cat, forming a big orange blotch. The same is true for those coding for black. (Tortoiseshells are completely black and orange, but calicos have white on their bellies, chests, and legs due to an another gene that causes piebalding—areas without any pigment in the skin and fur at all.)
The pattern is random, so that no two calicos or tortoiseshells are alike. Each is both an orange cat and a black cat, and there will never again be one like her. Identical twins don’t even have the same patches, because chance dictates which X-chromosomes are inactivated, and therefore which cells carry the orange trait and which ones code for black. The first cat to be cloned was a calico whose clone looked very different from her. A cell from Rainbow the calico became CC, who is dark gray (“blue,” in the official CFA terminology) and white. (Rainbow was a less common type of calico, in which the dark patches are not pure black but dark tabby gray, a pattern sometimes called caliby.) The implication: The cell that led to CC was one in which the black allele was active and the orange inactive.
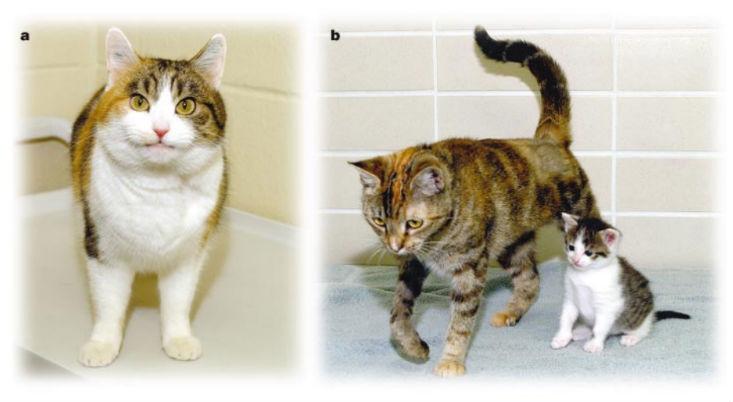
X-inactivation plays out differently in other parts of the body, so that some tissues have big patches with only maternal X or paternal X, and other tissues include each sprinkled in with the other. In a 2014 study with mice, Jeremy Nathans of Johns Hopkins University labeled Xs in either red or green depending on which of the two chromosomes was active. The result was stunning diversity of patterns: big patches of red and green in the intestinal lining, streaks in the smooth muscle of the gut, tiny stipples in the brain. As to what consequences there might be for the creature as a whole, there are as yet few answers.
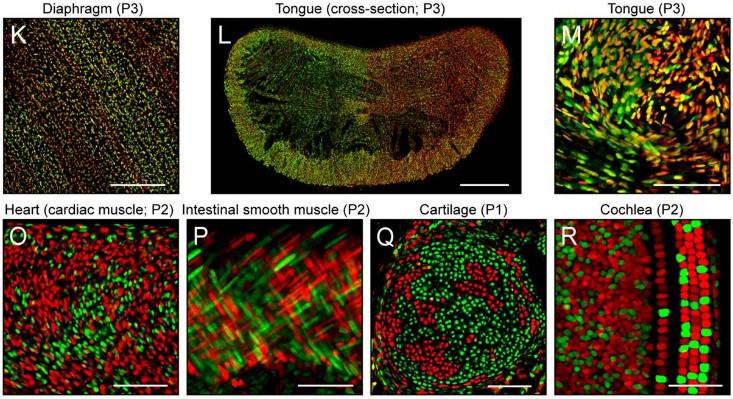
In general, the phenomenon of X-inactivation has been underappreciated and understudied, says Barbara Migeon, a professor at the Johns Hopkins Institute of Genetic Medicine. When Mary Lyon first proposed the idea of X-inactivation, Migeon was just beginning her fellowship in genetics, and she got hooked. “I was in the right place at the right time,” she says now. Ever since then, she has studied its mechanisms and consequences, and it has lead her to propose a theory: X-inactivation gives women an inherent genetic advantage.
The idea is basically that female mammals simply have more options, because the cells in their bodies are more diverse: They have two different X chromosomes with two different sets of gene variants. That greater range of possibility allows females to survive diseases that cripple or kill males, an effect that she explores in her 2007 book, Females are Mosaics. “We have an advantage in health as well as disease,” she says. “It’s the fact that we have heterogeneity for a lot of proteins, and can make new proteins that males don’t make.”
The female genetic advantage is obvious in the case of X-linked diseases, which are caused by a mutation on the X chromosome. Since males have only one X chromosome, if they have a mutation there, they have only that one mutated copy. With no good version to fall back upon, they get very sick or die. But women almost always have another copy of the gene that is normal and can compensate. With this backup system, they stay relatively healthy, or sometimes have no symptoms at all. Migeon puts it this way: A female is a composite of two intermingled cell populations that share gene products with one another. The result is an organism that is inherently more resilient.
One example is Fabry disease, a rare condition caused by a mutation in the GLA gene that disrupts the production of an enzyme called alpha-galactosidase A. Without functional enzymes, a type of fat accumulates in cells all over the body, leading to cataracts, strokes, kidney failure, and other serious symptoms.
A woman who inherits the mutation usually only has one bad copy; the good gene on her other X rescues her from that fate. Even though just half her cells produce functional protein, they share it with the sick cells or simply take over their job. A woman with a GLA mutation usually will have milder symptoms or symptoms that begin late in life. (Incidentally, this is also what maintains the mutation in the species: Women live long enough to pass the gene on to the next generation.) Duchenne muscular dystrophy works much the same way—with rare exceptions, only boys are affected, due to a mutation of a gene on the X chromosome.
Males die at higher rates than women at every age, from birth to old age—and even in utero and among infants. X-inactivation could be the reason.
Some X-linked genetic disorders are so severe that only women have them, because only they can survive long enough; males with the same mutation die before they are born or shortly after birth. Rett syndrome, which causes autism-like symptoms, is one of them. Incontinentia pigmenti, a mutation in the IKBKG gene that causes problems with the skin, eyes, and teeth, is also almost completely a female disease.
Migeon points out that men die at higher rates than women at every age, from birth to old age, a disparity that’s usually chalked up to male hormones, larger body size, or risk-taking behavior. But the survival disadvantage for men occurs even in utero and among infants, when these factors have little or no effect. X-inactivation could be the reason.
She proposes that the X effect goes beyond protection from disease; Migeon thinks that women likely have other biological advantages as well. If 20 percent of the roughly 1,100 genes of the two X chromosomes are functionally different from one another, on average, that potentially provides women with a whole new set of molecules and new cellular functions.
In the brain, for example, it’s entirely possible that this genetic diversity could give rise to cells that have slightly different capabilities, leading to networks that enable a broader range of responses or even new ways to process information. X-chromosome inactivation “may represent one of the more significant mechanisms by which individual differences in [brain] function are generated,” writes Nathans in the conclusion of his study on how X-inactivation manifested in different parts of the mouse body. Migeon puts it a different way. “We want to be equal to men, but we really are better,“ she quips.
So far, the evidence doesn’t quite prove that. But the study of the effects of X-inactivation is relatively new, and it’s plausible that having a broader repertoire of genes can confer a variety of as-yet-unknown biological advantages. Among New World monkeys, for example, X-inactivation enhances vision in females. The gene that controls the pigments in the monkey eye that make it possible to distinguish between different wavelengths of light is located on the X chromosome. In New World monkeys the gene has three alleles, meaning that while males can only have two of the three alleles and are restricted to dichromatic color vision, some female monkeys carry all three. Their world, like ours, is one of brilliant hues.
Kat McGowan is a contributing editor at Discover magazine and an independent journalist based in Berkeley, Calif., and New York City.