You might have learned in school that there are three phases of matter: solid, liquid, and gas. That is a useful simplification for young students, but there are in fact many, many more. In the past century or so, we’ve discovered that there are hundreds of distinct solid phases—some of which are used to build the silicon chips that run your computer. In addition, there are dozens of liquid crystal phases—some of which create the images on your laptop screen. And that’s before we even get to the really exotic stuff: quantum phases like superfluids, quark-gluon plasma, Bose-Einstein condensates, and the so-called “topological phases.”1
But before we get to that, let’s step back and discuss what we mean by the word “phase.” Like many fundamental concepts, phases are best explained by example. Let’s consider a glass of water with some ice cubes in it. The glass contains just one kind of substance: water. A whole lot of molecules of H2O.
Even though the molecules in the ice cubes are exactly the same as those in the surrounding water, there’s clearly a big difference between ice and liquid water. Most obviously, one is rigid, holding a defined shape, and one flows freely, taking the shape of its container. There are also differences in density, electrical conductivity, and a host of other physical properties. So we say that liquid water and ice are different phases of a single substance.2
How can it be that ice and water display such radically different behavior? You might be tempted to say that the difference is the temperature: Water is warm and ice is cold, and somehow this makes the water molecules behave differently. But at 0° C and normal atmospheric pressure, both ice and liquid water are stable—that is, they can exist indefinitely without changing.
So if temperature isn’t the key difference between ice and liquid water, then what is?
Thinking Big and Small
Scientists have long speculated about a connection between the human-scale (or macroscopic) properties of various materials, and the microscopic arrangement of the “pieces” of which those materials are made. One early attempt was made in 1611 by Johannes Kepler, in the delightfully titled, “The Six-Cornered-Snowflake: A New Year’s Gift.” Kepler argued that the hexagonal symmetry of snowflakes could be explained if ice was built out of tiny spheres packed together in regular hexagonal arrays.
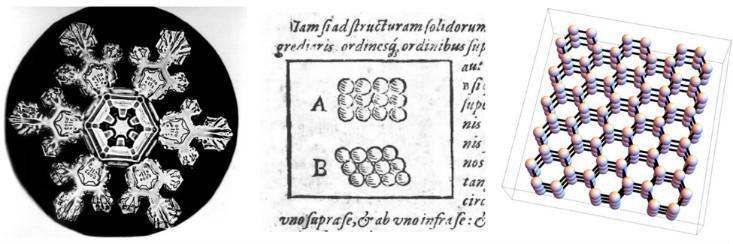
We now know that Kepler’s conjecture about the specific arrangement of atoms was slightly wrong—the arrangement he drew doesn’t correctly account for the 3D shape and complex interactions of water molecules. (In fairness to Kepler, chemistry hadn’t been invented yet, and the existence of atoms wouldn’t be proven conclusively until almost 300 years later.) Nevertheless, he was on the right track. The six-fold symmetry of snowflakes does come from the fact that the water molecules are arranged in a 3D structure with hexagonal symmetry.3
In the language of modern physics, Kepler conjectured that snow is a crystalline solid—a phase of matter which gets its properties because the atoms or molecules are packed into a regular array called a crystal lattice. Modern physicists use the word “crystal” to refer to the microscopic structure itself, regardless of whether or not a material looks to the eye like a diamond or a grain of salt or a snowflake. Thus, to a physicist, many metals and ceramics are crystals, because the atoms or molecules are arranged in a regular lattice—essentially, some 3D version of Kepler’s 1611 drawings.
So that explains the ice in the water glass. But what about the liquid water? In any substance above absolute zero (-273° C), the molecules are constantly moving around, bouncing off each other like billiard balls after a powerful break. The temperature of a substance is simply a measure of how fast the molecules are moving.
In a liquid, this random thermal motion is strong enough to overcome the forces between the molecules that hold the lattice in place. And without a lattice locking them in place, water molecules can move much more freely around one another. This explains why liquid water gives way when you push on it with your finger, but ice doesn’t. (Curiously, there are some substances, like window glass, that have a totally disordered microstructure, but still behave like solids at a macroscopic level. Understanding this behavior might be the most important open question in materials physics.)
Phases Fantastic
In 3D materials—like real ice, rather than Kepler’s 2D sketch—things can get complicated, because in three dimensions there are many different ways to arrange molecules in a regular array. There are, for instance, 17 known phases of ice4, the most recent of which was first created and studied by Italian researchers last year. The different phases occur under various conditions of temperature and pressure, and, while they are all solids, each has a different density, hardness, response to electric and magnetic fields, thermal conductivity, and so on. These differences in macroscopic behavior are the reason we say they are different phases. Under “normal” conditions—the conditions of temperature and pressure found on the surface of the earth—ice is usually in the form ice Ih (“ice one h”). This hexagonal form is responsible for the six-fold symmetry of snowflakes.
(The labeling of the various ices means, by the way, that there really is an ice IX. Luckily, at the temperatures and pressures on the surface of the earth, it’s much more fragile than the other ice nine—the stable phase that catastrophically renders the oceans solid in Kurt Vonnegut’s book Cat’s Cradle.)
And the story isn’t over yet, even for this most quotidian of substances. A number of yet-to-be-discovered phases of ice might exist in the ultra-high pressures at the center of giant gas planets like Uranus and Neptune. Under these extreme conditions—10 million atmospheres of pressure or more—water is predicted to form some pretty weird crystalline solid phases. These include a conducting metallic phase, which would look shiny, if you could somehow figure out how to polish it.
The In-Betweeners
The connection between micro-structure and macro-properties is a central idea in science and engineering and is used in the design of many kinds of materials. For instance, liquid crystals are the key components in our laptop, TV, and cellphone LCD (“liquid crystal display”) screens. Liquid crystals have molecules with an unusually long, rod-like shape. Because of this, these molecules can form a whole array of phases with properties that we don’t see in regular materials.
For instance, at high temperatures, the molecules are completely disordered: Each rod is positioned randomly, like the molecules in liquid water, and each rod is oriented in a random direction. This is called the isotropic phase. At lower temperatures, the molecules can shift into the nematic phase, where each one is positioned randomly, as in liquid water, but the molecules generally point in the same direction. Since there’s no lattice, the nematic phase can flow like a liquid, but, because all the rods are aligned, the phase has a particular kind of microscopic order. Hence the term “liquid crystal.”
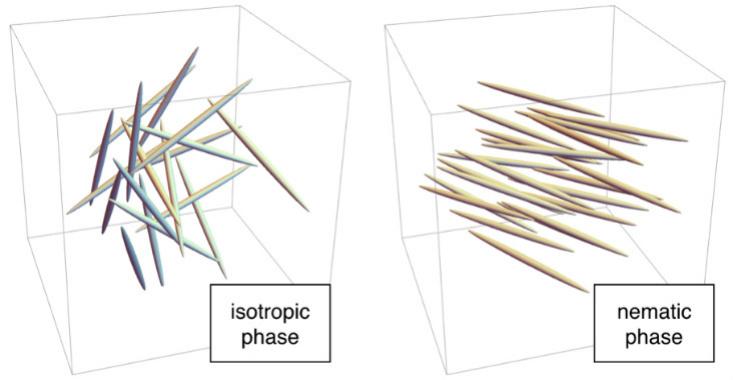
Luckily for all of us in the modern era, the nematic phase has unusual, direction-dependent properties. When placed in an electric field, the rod-shaped molecules tend to line up with that field. In LCD screens, molecules in the nematic phase behave as a polarizing filter: a device that allows incoming light to pass when the molecules are oriented one way (for example, pointing up-down), but not when they’re oriented in the perpendicular direction (left-right)5. In an LCD, tiny electrodes across each pixel create an electric field that controls the orientation of the molecules inside that pixel, and thus whether or it emits light6.
Modeling the Minuscule
It’s clear that micro-structure helps explain the properties of many materials we encounter and create. But can we actually see this microscopic ordering in real life? For regular substances, like ice or salt or metal, this is tricky, because atoms and molecules are so tiny. A water molecule, for instance, is less than a billionth of a meter across—much too small to see with a normal light microscope, and small enough that it’s challenging to observe even with the most modern microscopy techniques.
Luckily, atoms and molecules aren’t the only things that self-organize into phases. In the Chaikin lab at the New York University Center for Soft Matter Research, we use small, solid spheres to study phases of matter. These little spheres are called colloids, and they can be made out of a wide range of different materials, like glass, plastic, or metal. The colloids we use in our lab are around 3,000 times bigger than water molecules—comparable in size to a bacterium or the nucleus of an animal cell. For materials scientists, colloids are something of a “Goldilocks particle”—big enough to see with a microscope, but small enough to make phases that are in many ways analogous to regular materials, like ice or water.
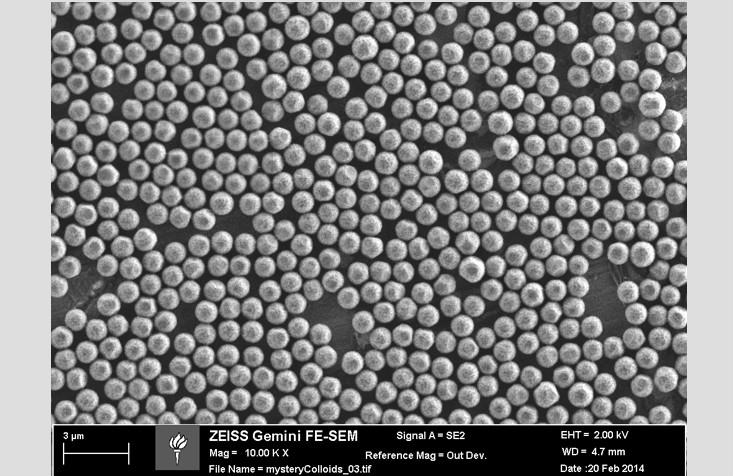
At NYU, we manufacture colloids that have a special coating that allows them to stick to water surfaces. When our particles stick to droplets of water, we can take beautiful microscope images like the ones shown below. In these images, we can see the microscopic structure of the different phases, and how that order leads to macroscopic properties like solidity. For instance, we are currently studying how the behavior of our particles changes when they are stuck to spherical droplets as opposed to flat surfaces like a still pond. Does being on a sphere help or hinder the formation of crystals and the emergence of solid-like behavior? Ultimately, this might help us to understand the properties of important structures that are spherical or have even more complex shapes. If, for instance, we knew more about the protein shells that protect the genes of HIV, we might be able to break those shells and render the virus harmless.
When Crystals Go Bad
One of the most important parts of learning about microscopic order is to understand when and how that ordering fails. For instance, if you look closely at a picture of colloids in a crystal lattice, you’ll notice that the ordering isn’t uniformly precise: We can observe imperfect or disturbed regions, which are called defects.
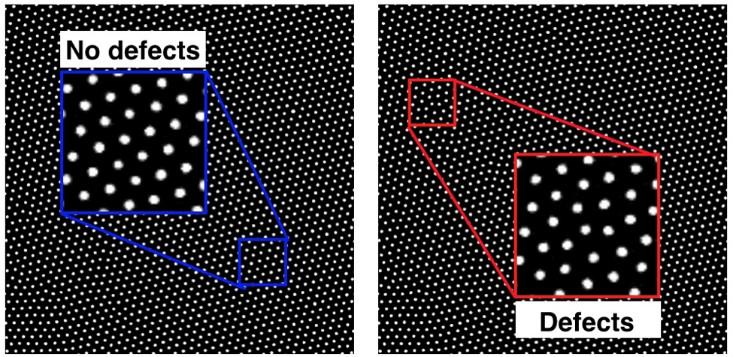
Similar defects occur in atomic and molecular crystals too, and they turn out to be important in lots of ways. For instance, they’re critical in determining the brittleness of a material—how much load it can bear before it breaks. Additionally, because crystal defects can affect the way a material conducts electricity, semiconductor manufacturers spend billions of dollars producing monocrystalline silicon—gigantic blocks of silicon with almost no defects. On the other hand, sometimes these microscopic blemishes are very desirable. Recent research has focused on how to control and manipulate defects in graphene to optimize it as a filter for water desalination, for instance.
At NYU, we use colloidal crystals to see how defects are created, move around, and interact with one another. As with phase behavior, studying what happens in our colloidal crystals can help us to understand defects in other materials, too. As with many things in life, sometimes the imperfections are the most interesting parts of all.
Colm P. Kelleher received his PhD in Physics at New York University. He is one of the authors of Phases of Matter Coloring Book (Green Frog Publishing), which will be published in 2017.
Footnotes
1. The term “state of matter” is sometimes used interchangeably with “phase of matter,” and, like many fundamental concepts in science, neither term has a unique, universally agreed-upon definition.
2. In fact, water is the only substance that we humans regularly encounter in three different phases. This is just one of the many reasons water is a very special material.
3. There are also triangular snow crystals, but nobody is quite sure why.
4. There are roughly 17 phases of ice. As with many questions of taxonomy, it depends on how you count.
5. This is true if the incoming light is linearly polarized. In a phone or laptop display, this is achieved by putting a polarizing filter between the light source and the screen.
6. This description refers specifically to “in-plane switching” LCD’s (IPS-LCD’s). In an IPS-LCD, a lighting panel behind the screen emits white light of uniform brightness. The screen itself is composed of a mesh of pixels, each containing three sub-pixels. The sub-pixels contain a thin layer of nematic-phase rods. Tiny electrodes placed on either side of each sub-pixel control the orientation of molecules, and thus whether or not light will pass through that sub-pixel. The three sub-pixels are coated with red, blue and green color filters, thus allowing the pixel to express any color. Other designs include the “twisted nematic” LCD (TN-LCD) which uses an even more exotic “twisted nematic” liquid crystalline phase to achieve a similar result. Check out the video here for an in-depth explanation of the TN-LCD in detail, or check HowStuffWorks for a good overall explanation of LCDs.
7. These pictures were taken with a type of microscope called a confocal microscope. In confocal microscopy, fluorescent dye is embedded in the sample, and a laser is used to make the dye glow. In these pictures, we’re seeing the emitted glow from the colloids themselves.
WATCH: The UC Berkeley chemist Richard Saykally on why 17 forms of ice exist.
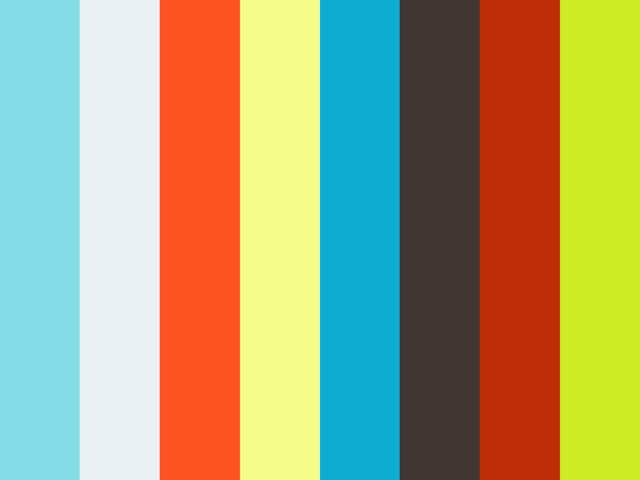