In their attempts to pin down the meaning of “life,” scientists and philosophers have offered dozens of definitions. Lately, researchers more or less agree that, for something to be alive, it has to be able to reproduce and evolve by natural selection.
While these attempts might be getting us incrementally closer to understanding what life on Earth is, and what life elsewhere could be, none has proven particularly helpful in deciphering how life arose. So far, the most fruitful approach has involved creative experiments. These include ones aimed at finding a plausible path, based on what we know about the early Earth’s environment, by which rudimentary chemistry could have become simple biology. Not surprisingly, early attempts to do this encountered a seemingly insurmountable obstacle: The inability to determine what came first—the power to speed up critical chemical reactions, or the power to carry information.
Take, for instance, the main molecular components of life—the nucleic acids (RNA and DNA) and proteins. RNA and DNA are responsible for storing life’s operating instructions and all of the information that gets passed on from one generation to the next. Proteins are life’s workhorses, carrying out most of the biochemical functions of a cell. But making proteins requires the information encoded in RNA and DNA, while at the same time assembling RNA and DNA themselves requires proteins!
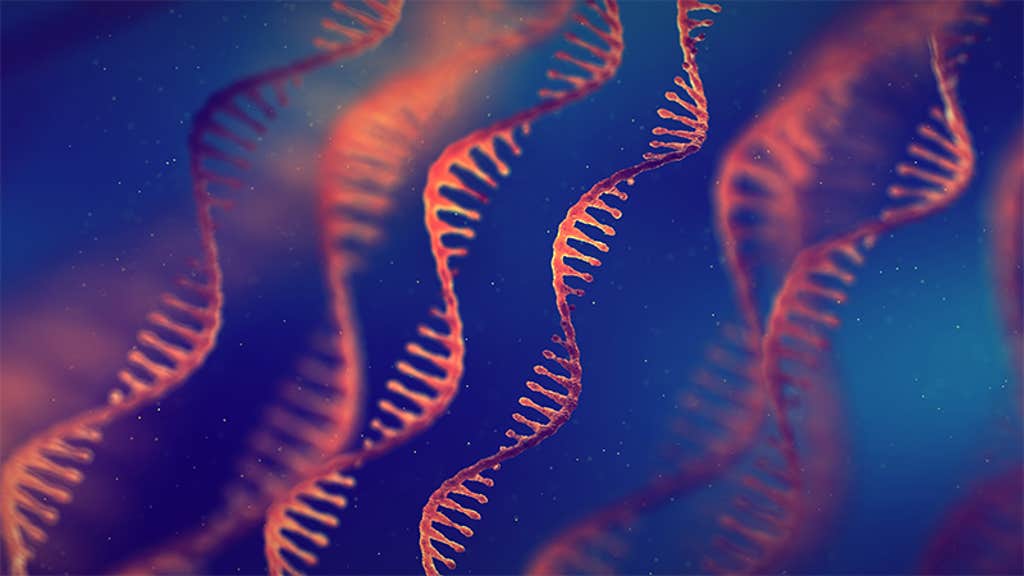
Similarly, it’s protein enzymes that ignite metabolism, the chemical processes within cells that allow them to use nutrients and energy sources; yet what creates those enzymes are biochemicals that owe their existence to metabolism! How could one spin up without the other existing first?
For many years, this apparent circle of causes has been a real “chicken or egg” dilemma and convinced many scientists that life could only have emerged through an almost miraculous chemical accident. How else, these scientists wondered, could a chaotic mixture of chemical elements suddenly transform into biological life? As we explore in our new book, Is Earth Exceptional?: The Quest for Cosmic Life, research in the last two decades has succeeded in finding a creative way out of this labyrinth.
The first hints of a potential solution to this problem were discovered in the late 1960s. Robert W. Holley, a biochemist at Cornell University, worked out the sequence and chemical structure of a particular RNA molecule, known as transfer RNA or tRNA. Then biophysicists Aaron Klug and Alexander Rich followed that up by untangling the folded architecture of tRNA. To everybody’s enormous surprise, this RNA’s structure looked more like a protein than DNA, with intricate folds—nothing like the rather stiff, featureless, ladder-like double helix of DNA.
Chemist Leslie Orgel and molecular biologist Francis Crick immediately realized what this meant. They suggested that RNA might have played the role of a protein enzyme during the origin of life—sparking chemical reactions as well as carrying information. Orgel, in the late 1960s, boldly speculated that early life on Earth did not involve either DNA or proteins, only RNA.
How could a chaotic mixture of chemical elements suddenly transform into biological life?
Even with Orgel’s impeccable reputation, the idea that an RNA sequence could both store information and accelerate chemical reactions (at the time considered to be something only protein enzymes could do) was hard to accept. But scientists had no choice once molecular biologist Sidney Altman and chemist Thomas Cech made the stunning (and Nobel Prize-winning) discovery in the early 1980s that RNA could indeed act as an enzyme—and potentially start up its own replication.
This solved the incredibly thorny “which came first, the chicken or the egg?” conundrum. The implication was astounding: The origin of life might simply come down to the origin of a self-replicating RNA molecule.
Where does that leave proteins and DNA? They could have evolved later, with the advantages of being able to catalyze reactions more efficiently and storing information more robustly. This tantalizing concept of a simpler time in the history of life on Earth, with RNA alone being both the “chicken” and the “egg” was popularized by biochemist Walter Gilbert in the pithy catchphrase “the RNA world.” RNA molecules, housed inside protocells much less intricate than the cells we find in life today, could have been playing all the major roles, acting as both the carrier of genetic information and as functional molecules.
Even after the ingenious idea of the RNA world, understanding how simple primordial protocells could grow, replicate, and divide required a revolution in thinking. After all, those cells probably consisted only of a simple membrane, most likely composed of fatty acids, that housed short pieces of RNA. A major difference between those early protocells—the precursors of the microorganisms we see now—and cells today is that ours have in their interiors and in their membranes a formidable protein apparatus. This machinery enables and controls the transport of nutrients and water across the membrane.
This is the crucial realization that researchers had to come to grips with: Whereas today’s cells rely on their own internal biochemistry to do their work, protocells in the RNA world had to rely on their surroundings for nourishment—and it was fluctuations outside primordial protocells that drove the processes of protocell growth and division.
The origin of life might simply come down to the origin of a self-replicating RNA molecule.
Take one dramatic example: What happens when a protocell has multilayered boundaries? When supplied with additional fatty acid molecules, the outermost membrane sprouts a tube that gets thicker and longer, and as the layered membranes exchange material, the entire protocell becomes a long, fragile, multilayered filament. This makes cell division easy. Even weak shear forces, such as those exerted on the surface of a lake by the wind, can fragment such a filament into “daughter” protocells, while retaining precious contents (such as RNA) inside the progeny.
What in the world was happening billions of years ago to allow the first protocells to appear? There has never been a more exciting time to seek answers to this question. It is now clear that to have a chance at solving this mystery—as well as whether the origin of life was inevitable or a fluke—scientists will have to imagine what strange landscapes on Earth could have given birth to the building blocks of RNA, proteins, and cell membranes.
This is already proving fruitful. In 2009, researchers identified ways in which two of the four nucleotides of RNA could have formed on the early Earth. Given the fact that the current scenario is that life on Earth started with RNA, this means that we are, in some sense, halfway there. Even more remarkably, researchers have shown that precisely the same chemistry could have also produced 12 out of the 20 amino acids—the building blocks of proteins—involved in all life forms on Earth. In other words, we now have at least part of a plausible pathway from chemistry to biology.
These advances in prebiotic chemistry, together with our growing understanding of the early Earth environments that could have fostered this chemistry—like soda lakes or impact crater lakes—are bringing us closer to a picture of how life itself might have emerged on the surface of our newly formed planet.
Lead image: Paolo Farinella / Shutterstock