The name of the image—the “Flammarion engraving”—may not ring a bell, but you’ve seen it many times. It depicts a traveler wearing a cloak and clutching a walking-stick; behind him is a varied landscape of towns and trees; surrounding all is a crystalline shell fretted with countless stars. Reaching the edge of his world, the traveler pushes through to the other side and is dazzled by a whole new world of light and rainbows and fire.
The image was first published in 1888 in a book by French astronomer Camille Flammarion. (The original engraving was black and white, although colorized versions now abound.) He notes that the sky does look like a dome on which the celestial bodies are attached, but impressions deceive. “Our ancestors,” Flammarion writes, “imagined that this blue vault was really what the eye would lead them to believe it to be; but, as Voltaire remarks, this is about as reasonable as if a silk-worm took his web for the limits of the universe.”
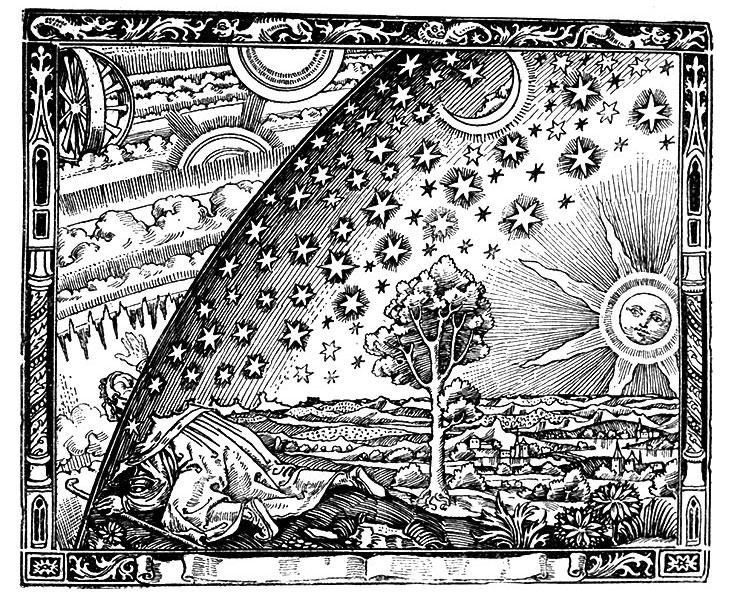
The engraving has come to be seen as a symbol of humanity’s quest for knowledge, but I prefer a more literal reading, in keeping with Flammarion’s intent. Time and again in the history of science, we have found an opening in the edge of the known world and poked through. The universe does not end at the orbit of Saturn, nor at the outermost stars of the Milky Way, nor at the most distant galaxy in our field of view. Today cosmologists think whole other universes may be out there.
But that is almost quotidian compared to what quantum mechanics reveals. It is not just a new opening in the dome, but a new kind of opening. Physicists and philosophers have long argued over what quantum theory means, but, in some way or other, they agree that it reveals a vast realm lying beyond the range of our senses. Perhaps the purest incarnation of this principle—the most straightforward reading of the equations of quantum theory—is the many-worlds interpretation, put forward by Hugh Everett in the 1950s. In this view, everything that can happen does in fact happen, somewhere in a vast array of universes, and the probabilities of quantum theory represent the relative numbers of universes experiencing one outcome or another. As David Wallace, a philosopher of physics at the University of Southern California, put it in his 2012 book, The Emergent Multiverse, when we take quantum mechanics literally, “the world turns out to be rather larger than we had anticipated: Indeed, it turns out our classical ‘world’ is only a small part of a much larger reality.”
This array of universes seems, at first glance, to be very different from the one that cosmologists talk about. The cosmological multiverse grew out of models that seek to explain the uniformity of the universe on scales larger than galaxies. The putative parallel universes are distant, distinct regions of spacetime, the result of their own local big bangs, evolving from their own bubbles of quantum foam (or whatever it is that universes sprout from). They are out there in roughly the same way that galaxies are—you could imagine getting in a starship and traveling to them.
Everett’s many worlds, in contrast, are down here. The concept emerged from efforts to understand the process of laboratory measurement. Particles leaving trails in cloud chambers, atoms deflected by magnets, hot objects emitting light: it was these sorts of hands-on experiments that motivated quantum theory and the search for a coherent interpretation. The quantum “branching” that occurs during a measurement gives rise to new worlds that overlap with the space where we live.
And yet these two kinds of multiverses have much in common. We can visit either sort only in our mind’s eye. Try as you might to reach another bubble universe in your starship, the intervening space would expand faster than you could possibly cross it; bubbles are thus cut off from one another. Likewise, we are by our very nature blind to other universes in the quantum multiverse. These other worlds, though real, remain forever out of view.
Moreover, although the quantum multiverse was not developed for cosmology, it is peculiarly well suited to it. In conventional quantum mechanics—the Copenhagen view, embraced by Niels Bohr and his collaborators—one has to distinguish between the observer and the thing being observed. That’s fine for standard laboratory physics. The observer is you, and the experiment is the thing you’re observing. But what if the object under investigation is the entire universe? You can’t get “outside” the universe in order to measure it. The many-worlds interpretation makes no such artificial distinctions. In a new paper, Caltech physicist Sean Carroll, together with graduate students Jason Pollack and Kimberly Boddy, directly applies the many-worlds interpretation to creation of universes in the cosmological multiverse. “Everything that’s very wishy-washy in conventional quantum mechanics becomes, in principle calculable [in the Everett view],” Carroll says.
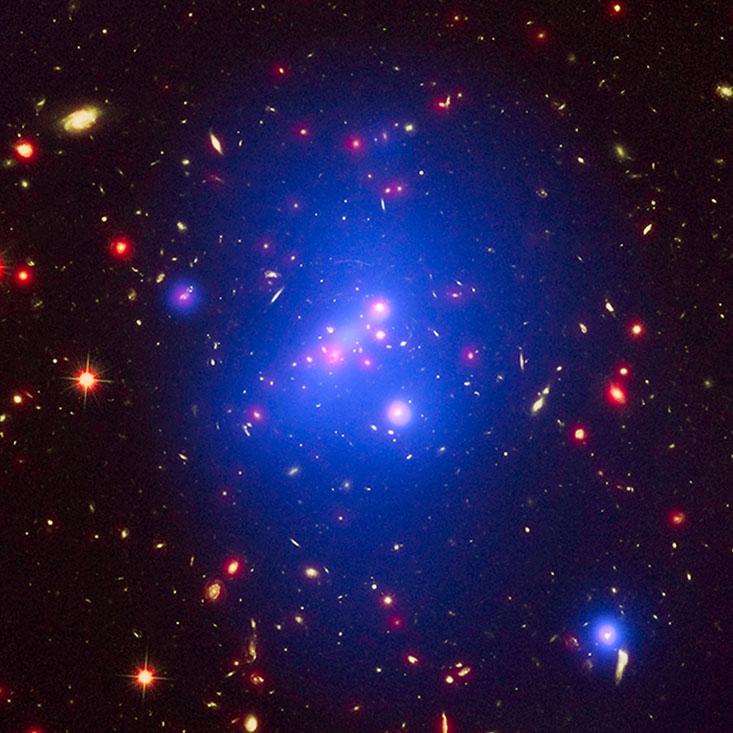
Finally, the two kinds of multiverse make identical predictions for our observations. The only difference is that they situate the possible outcomes in different places. Carroll sees an equivalence between “the cosmological multiverse, where different states are located in widely separated regions of spacetime, with a localized multiverse, where the different states are all right here, just in different branches of the wave function.”
MIT cosmologist Max Tegmark made this idea concrete during a talk he gave in 2002, which evolved into his 2014 book, Our Mathematical Universe. He describes several “levels” of multiverse. Level I simply refers to very, very distant regions of our own universe. Level III is his term for the quantum many worlds. (He also has levels II and IV, but we don’t need to worry about them here.) To see the similarity between levels I and III, you have to think about the nature of probability. If something can have two different outcomes, you only see one of them, but you can be sure that the other one has also happened—either in some other part of a giant universe or in a parallel world right here. If space is sufficiently large and filled with matter, events that occur here on Earth will also occur elsewhere, as will every possible variation of those events.
The reason quantum events are uncertain is that we are uncertain about where we are.
For instance, suppose you do an experiment in which you fling an atom at a pair of magnets. You will see it veer toward the lower magnet or the upper magnet with a 50-50 probability. In the many-worlds view, there are two worlds overlapping within your laboratory. In one, the atom goes up; in the other, it goes down. In the cosmological multiverse, there are other universes (or parts of our universe) where an identical twin of Earth has formed and where a humanoid creature performs the same magnet experiment, but gets a different result. Mathematically these two situations are identical.
Not everyone accepts the multiverse, let alone that varieties of multiverse are similar. But, keeping in mind that these ideas are still tentative, let’s see where they take us. They suggest a radical idea: that the two multiverses may not, in fact, be distinct—that the many-worlds view is the same as the cosmological multiverse. If they seem different, that is because we have been thinking about reality in the wrong way.
Stanford physicist Leonard Susskind suggested this equivalence in his 2005 book, The Cosmic Landscape. “The many-worlds view of Everett seems, at first, to be quite a different conception than the eternally inflating megaverse,” he writes (using his preferred term for the cosmological multiverse). “However, I think the two may really be the same thing.” In 2011 he and Berkeley physicist Raphael Bousso co-authored a paper in which they state flatly that the two are the same. They argue that the only way to make sense of the probabilities associated with quantum mechanics and of the phenomenon of decoherence—in which the theory gives rise to our classical categories such as positions and velocities—is by applying the many-worlds picture to cosmology; the natural result, they claim, is a cosmological multiverse. The same year, Yasunori Nomura of the University of California in Berkeley made a similar case, publishing a paper that, he says, “provides a fully unified treatment of quantum measurement processes and the multiverse.” Tegmark argues along similar lines in a 2012 paper co-authored with Anthony Aguirre of the University of California at Santa Cruz.
On this view, the quantum many worlds aren’t down here, but out there. The quantum wave function, Tegmark writes, doesn’t describe “some funky imaginary ensemble of possibilities for what the object might be doing, but rather the actual spatial collection of identical copies of the object that exist in our infinite space.”
The key, Bousso explains, is to think carefully about your point of view. Imagine taking a God’s-eye view of the multiverse in which you see all of the possibilities unfolding at once. There is no probability; everything happens with certainty, in some location. But from our own limited perspective, rooted here on planet Earth, various events unfold with various probabilities. “We trade a global picture, in which everything happens somewhere but nobody can see it all—for a local picture, where you have one patch, that could be in principle explored,” Bousso says.
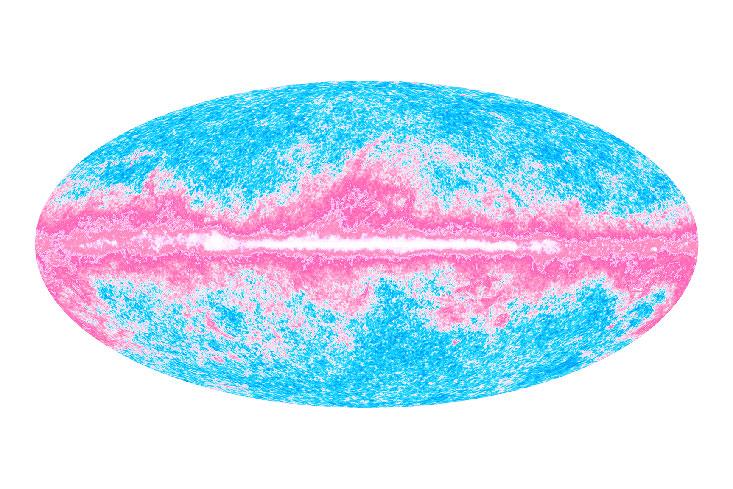
To navigate from the global to the local, we need to slice the universe so as to divide the measurable from the unmeasurable. The measurable part is our “causal patch,” as Bousso calls it. It is the sum of all that will ever be able to affect us—not just the universe that is observable today, but the region of space that will be accessible to our distant descendants. Having mentally sliced off our causal patch from the rest of spacetime, we can figure out what observations we are able to make, and the result is old-fashioned quantum mechanics.
From this perspective, the reason that quantum events are uncertain is that we are uncertain about where in the multiverse we are. In an infinite space, there are an infinite number of creatures that look and behave like you in every respect. A classic New Yorker cartoon gets to the crux of the matter. We see a gaggle of penguins—all identical—on an expanse of ice. One of the penguins asks, “Which one of us is me?”
The poor penguin might have some hope of triangulating its position relative to nearby ice floes, but in the multiverse we have no such reference points, so we can never distinguish among our multiple selves. David Deutsch—a physicist at Oxford and, like Carroll and Tegmark, a staunch supporter of many worlds—writes in his book The Fabric of Reality: “To assume that it is physically meaningful to ask which of the identical copies is me, is to assume that there is some frame of reference outside the multiverse, relative to which the answer could be given—‘I am the third one from the left…’ But what ‘left’ could that be, and what does ‘the third one’ mean?” There is no “view from outside the multiverse.”
Parallel universes are not out there in space, but elsewhere along our own timeline.
At its heart, Tegmark argues, the notion of probability in quantum mechanics simply reflects “your inability to self-locate in the Level I multiverse, i.e., to know which of your infinitely many copies throughout space is the one having your subjective perceptions.” In other words, events seem probabilistic because you’re never quite sure which you is you. Instead of being unsure of which way an experiment will unfold, it unfolds every way; you’re simply unsure of which “you” will observe which outcome.
For Bousso, the mathematical success of this approach is enough, and he prefers not to lose sleep over how one might interpret the deeper significance of these merged multiverses. “Ultimately, the only thing that matters is what predictions your theory makes and how they compare to observations,” he says. “The regions beyond our [cosmological] horizon are not observable, and neither are branches of the wave function that we didn’t end up in. Either way they are just tools we use to do the calculation.”
But such an instrumentalist view of physical theory strikes many as unsatisfying. We still want to know what it all means—how a reading on a laboratory dial could betray the existence of infinite bubbles of spacetime. Massimo Pigliucci, a philosopher of science at the City University of New York, says, “[If] you’re really talking about the universe somehow splitting, then you better give me a good account of how exactly this is happening, and where exactly are these [other worlds].”
Perhaps one way to make sense of the connection between breeds of multiverse is that our conventional views of time and space are in need of an update. If the multiverse is both out there and down here, perhaps that is a sign that our categories of “there” and “here” are failing us.
A system might not live in a single space, but in multiple spaces simultaneously—we would call such a system a multiverse.
Nearly two decades ago, Deutsch argued in The Fabric of Reality that the multiverse invites a new conception of time. In daily life and even in physics, we presuppose the existence of something like a Newtonian ever-flowing time. The multiverse is commonly described as a structure that unfurls in time. In actuality, time doesn’t flow or pass, nor do we progress through it in some mysterious way. Time is the means by which we define motion; it cannot itself move. So, the multiverse does not evolve. It just is. Deutsch writes: “The Multiverse does not ‘come into existence’ or ‘cease to exist’; those terms presuppose the flow of time.”
Instead of thinking the multiverse as playing out within time, Deutsch thinks we should think of time as playing out within the multiverse. Other times, he says, are just special cases of other universes. (Independent physicist Julian Barbour also explored this idea in his 1999 book, The End of Time.) Some of these other universes, Deutsch says, bear such a close resemblance to our own—our “now”—that we interpret them as part of our universe’s story rather than as separate universes. To us, they are not out there in space, but along our timeline. Just as we can’t experience the multiverse all in one go, we can’t experience this infinite array of moments at once; instead, our experience reflects our perspective as embedded observers, inhabiting single moments. In passing from the global to the local view, we recover time’s familiar trappings.
Our notions of space, too, might be revamped by the multiverse. “Why does the world look classical?” Carroll asks. “Why are there four dimensions of spacetime?” Carroll, who has blogged about the question of merging multiverses, admits that Everett doesn’t answer those questions, “but it gives you a framework in which you can ask them.”
He and others believe that space is not fundamental, but instead an emergent phenomenon. But what does it emerge from? What kind of “stuff” actually exists? For Carroll, the Everettian picture provides a remarkably simple answer to this question. “The world is a wave function,” says Carroll. “It’s an element of Hilbert space. That’s it.”
Hilbert space is the mathematical space associated with the quantum wave function. It is an abstract representation of all possible states of a system. It’s a bit like regular Euclidean space, but with a variable number of dimensions depending on how many states the system is allowed to have. A qubit—the fundamental data unit in quantum computers, which can be either ‘0’ or ‘1’ or some combination thereof—has a two-dimensional Hilbert space. A continuous quantity such as position or velocity corresponds to an infinite-dimensional Hilbert space.
Normally physicists start with a system that lives in real space and deduce its Hilbert space, but Carroll thinks you can invert that process. Imagine all the possible states of the universe and work out what type of space the system must be living in—if, indeed, it lives in space at all. The system might not live in a single space, but in multiple spaces simultaneously, and we would call such a system a multiverse. This view “is a very natural fit to the idea that spacetime is emergent,” Carroll says.
Some people—especially philosophers—balk at this approach. Hilbert space might be a perfectly legitimate mathematical tool, but that doesn’t mean we live in it. Wallace, a strong supporter of the many-worlds view, says that Hilbert space is not a literally existing structure, but a way of describing actual, physical stuff, be it strings, particles, fields, or whatever else the universe is ultimately made of. “There’s a metaphorical sense in which we live in Hilbert space, but maybe not a literal sense,” he says.
Hugh Everett didn’t live long enough to witness the renewal of interest in his version of quantum mechanics. He died of a heart attack in 1982, at the age of 51. A staunch atheist, he was certain that this was the end; his wife, following his instructions, threw his ashes out with the trash. His message, however, may finally be taking root. It can be summed up in four words: Take quantum mechanics seriously. When we do so, we find that the world is—surprise!—larger and richer than we had imagined. Just as Voltaire’s silkworm saw only his web, we see only a small sliver of the multiverse, but, thanks to Everett and those who followed in his footsteps, we may yet slip through the crack in the crystalline shell, “where the earth and the heavens meet,” and glimpse what lies beyond.
Dan Falk is a science journalist based in Toronto. His books include The Science of Shakespeare, In Search of Time, and Universe on a T-shirt. @DanFalk
Lead image: Multi-surfaced kaleidoscope-like mirrored entrance at OmoHara shopping center in Tokyo. Jeremy Sutton-Hibbert/Getty Images.
This article was originally published on Nautilus Cosmos in January 2017.